X-ray diffraction (XRD) is a versatile technique used commonly in the field of nanotechnology to characterize and acquire accurate information regarding the composition, crystal structure, and crystalline grain size of nanoparticles.
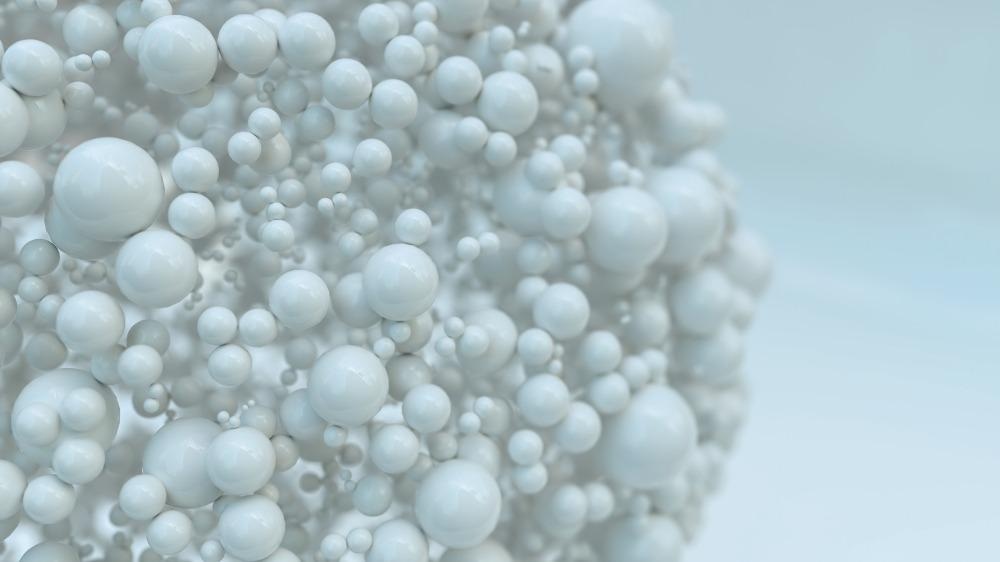
Image Credit: Crevis/Shutterstock.com
XRD method is best suited for powdered samples, which are freshly prepared from drying the samples’ respective colloidal solutions. From the XRD equipment, the peaks’ position and intensity of a sample are compared with the patterns from various diffraction database references, which assists in quantifying the composition of nanoparticles (Mourdikoudis, Pallares, & Thanh, 2018).
The working principle of the XRD method involves the scattering of X-rays due to the revolution of electrons in the atom’s nucleus when the rays strike on the nanoparticles. The scattered X-rays are reflected in various directions, which cause interference patterns. These patterns are either destructive or constructive (Fultz & Howe, 2013) but only the scattered X-rays that undergo constructive interaction result in diffraction.
In a nanoparticle, constructive interference results when two waves are moving in phase with each other, and destructive interference results from out-of-phase movement. The atomic-scale arrangement and the diffraction are strongly and inversely correlated: atoms having shorter periodic arrangements show greater diffraction angles and vice versa (Fultz & Howe, 2013).
Characterization of Nanoparticles Using XRD
The characterization of the nanoparticles through XRD requires a source, a detector, and a sample.
Thin films of nanoparticles are prepared for XRD analysis, which involves drop-casting of the nanoparticle onto a suitable low-background substrate. Nanoparticle samples that have the orientation of interest are placed on a suitable goniometer, where several reflections are evaluated. However, a sample of random orientation may only require a quick scan through a 2θ diffraction angle.
Nanoparticles characterization from XRD begins with the identification of the sample material’s phase; the crystal type in the sample is determined through a search-match process, which is done in the regions where the peaks of high intensities are observed.
Various XRD peak patterns are the results of various atom arrangements. In an XRD technique, the interference occurs when the light of a designated wavelength illuminates a periodic structure having a predefined spacing. The XRD principle follows Bragg’s law, (n*λ = 2*d*sinθ), where λ, n, d, and θ refers to X-ray wavelength, integer, atomic plane spacing, and diffraction half-angle, respectively. As a result, information regarding the sample’s crystal defects, crystal size, crystalline phase, shape anisotropy, strain, texture can be obtained from the evaluation of the diffraction peaks’ width, shape, and position (Widjonarko, 2016).
Importance of Nanoparticle Characterization
The characterization of nanoparticles has become increasingly important because they are synthesized from several mechanical or chemical routes (Koczkur, Mourdikoudis, Polavarapu, & Skrabalak, 2015), and they have huge potential technological applications and academic research interests across several fields.
Since nanoparticles have a large surface area-to-volume ratio, their properties are significantly altered with size, which makes the characterization an important step to understand their properties at different molecular levels.
Other properties, such as texture, strain, shape anisotropy, crystalline phase, crystal defects, and crystal size impact the chemical, electronic, mechanical, and optical attributes of the nanoparticles.
Without the proper characterization, the applicability of the specific nanoparticle would meet an immense challenge (Thanh, Maclean, & Mahiddine, 2014).
Key Research
In their research, (Wu, et al., 2015) focused on investigating the eco-friendly gold nanoparticles and used XRD to confirm the single crystallinity nature of APM-AuNPs, demonstrating the peak corresponding to the (111) plane.
In other research, (Li, et al., 2013) synthesized nanostructures of a copper telluride of varying shapes, such as rods, plates, and cubes, and their XRD experiment discovered that the relative intensities were correlated with the nanoparticles’ shapes.
Another group, (Upadhyaya, Parekh, & Pandey, 2016) applied the X-ray line broadening technique and determined the magnetite nanoparticles’ average crystallite size, which they discovered was in the 9–53 nm range. Their findings implied the crystallite’s lattice strain and size impacted the XRD peak broadening. The team also found that the technique is not appropriate for differentiating the boundaries between the two crystallite lattice, and the real transmission electron microscopy (TEM) size of a few samples calculated from the Scherrer formula were bigger than 50-55 nm.
Future Developments
Nanoparticles are usually produced in low quantity on the lab scale, making them challenging for sample preparation and screening.
In the future, improvement in the accuracy and resolution of XRD should be of primary focus. The synthesis of nanoparticles that have diverse physio-chemical, electronic, mechanical, and optical properties have huge demand and they require credible and precise protocols for characterization. In practice, the current characterizations tools for nanoparticles bear some unavoidable errors and compared to that of bulk materials, are difficult to analyze. A comprehensive approach to characterization is appreciated and the best results may yield if XRD is complemented with other instrumental methods.
References and Further Reading
Fultz, B., & Howe, J. (2013). Transmission Electron Microscopy and Diffractometry of Materials. Springer. doi:10.1007/978-3-642-29761-8
Koczkur, K., Mourdikoudis, S., Polavarapu, L., & Skrabalak, S. (2015). Polyvinylpyrrolidone (PVP) in nanoparticle synthesis. Dalton Transactions. doi:10.1039/C5DT02964C
Li, W., Zamani, R., Gil, P., Pelaz, B., Ibáñez, M., Cadavid, D., . . . Alvarez-Puebla, R. (2013). CuTe nanocrystals: shape and size control, plasmonic properties, and use as SERS probes and photothermal agents. J Am Chem Soc. doi:10.1021/ja401428e
Mourdikoudis, S., Pallares, R., & Thanh, N. (2018). Characterization techniques for nanoparticles: comparison and complementarity upon studying nanoparticle properties. Nanoscale. doi:10.1039/C8NR02278J
Thanh, N., Maclean, N., & Mahiddine, S. (2014). Mechanisms of Nucleation and Growth of Nanoparticles in Solution. Chem. Rev. doi:10.1021/cr400544s
Upadhyaya, S., Parekh, K., & Pandey, B. (2016). Influence of crystallite size on the magnetic properties of Fe3O4 nanoparticles. Journal of Alloys and Compounds. doi:10.1016/j.jallcom.2016.03.279
Widjonarko, N. (2016). Introduction to Advanced X-ray Diffraction Techniques for Polymeric Thin Films. Coatings. doi:10.3390/coatings6040054
Wu, S., Yan, S., Qi, W., Huang, R., Cui, J., Su, R., & He, Z. (2015). Green synthesis of gold nanoparticles using aspartame and their catalytic activity for p-nitrophenol reduction. Nanoscale Research Letters. doi:10.1186/s11671-015-0910-7
Disclaimer: The views expressed here are those of the author expressed in their private capacity and do not necessarily represent the views of AZoM.com Limited T/A AZoNetwork the owner and operator of this website. This disclaimer forms part of the Terms and conditions of use of this website.