The origin of the matter-antimatter asymmetry in the universe is one of the most important outstanding questions in modern physics. This asymmetry occurred way back in the earliest moments of the history of our universe. To find the answer to that question, scientists push the boundaries of current knowledge by using the latest laser technology to create, contain, and study antimatter as a way of learning about the early evolution of the universe.
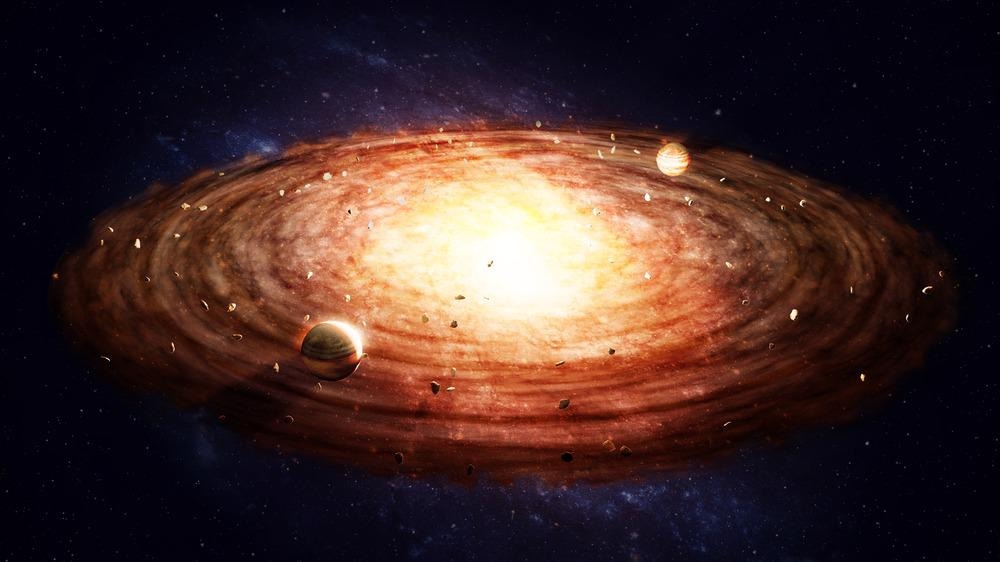
Image Credit: Mopic/Shutterstock.com
Since its development in the early 1970s, physicists use the Standard Model of particle physics to successfully describe and predict most of the observed high-energy physics phenomena and elementary particle interactions. In almost every process ever observed or predicted by the Standard Model, regardless of whether it’s taking place in a particle physics laboratory or the far reaches of the universe, the creation of an elementary particle is accompanied by the creation of its antiparticle. Conversely, when a particle interacts with its antiparticle, the two annihilate.
Antimatter Could Provide Valuable Insights into the Evolution of the Universe
One of the all-time great puzzles in modern physics is why our universe is made almost entirely of matter. Decade-long astronomical observations and high-energy cosmic ray studies unequivocally indicate that our universe contains more matter than antimatter, raising the question of how lots of matter was created during the Big Bang without an equivalent amount of antimatter.
In the Standard Model, the physical properties of a particle, such as an electron, appear to be equal and opposite to its antimatter counterpart, the positron. For example, electrons and positrons have the same mass but opposite electrical charges. Many scientists hypothesize that finding previously unknown tiny differences between particles and antiparticles that lay beyond the Standard Model could shed light on the causes for the matter-antimatter asymmetry in the universe.
Light Creates Elementary Particles
The idea that pairs of particles and antiparticles, such as electron-positron or proton-antiproton pairs, can be created when photons collide is central to modern physics. The concept illustrates that the energy and mass are equivalent, as demonstrated by Einstein with his famous equation E=mc2. However, direct observation of the phenomenon is still elusive since it requires the collision of photons with extremely high energies.
One of the options to create many particle-antiparticle pairs is to use high-power laser beams. High-intensity laser pulses ionize normal matter and accelerate the resulting free electrons to relativistic energies. This effect allows the laser pulse to interact with the plasma and induce strong currents capable of sustaining magnetic fields that are hundreds of kT (kiloTesla) in strength.
Multiple experiments involving plasma irradiated by high-intensity lasers have already improved our understanding of laser-driven electron acceleration, nuclear fusion, and star formation and evolution.
Harnessing the Laser Power to Create Antimatter from Vacuum
In the past decade, researchers have discovered that such intense magnetic fields can generate ultra-high-energy γ-rays inside the laser-irradiated plasma.
An international group of physicists from the University of California at San Diego and Extreme Light Infrastructure-Nuclear Physics facility in Romania devised an experiment that uses a tightly focused high-power laser with a peak power density of 5×1022 W/cm2 to generate a magnetic field as strong as that of a neutron star (100 million times stronger than Earth's magnetic field).
The laser pulse and the magnetic field exist only for 100 femtoseconds (a femtosecond is 10-15 seconds) but that is sufficient to create intense beams of γ-ray radiation. The colliding γ-ray beams are projected to create between 10,000 and 100,000 particle-antiparticle pairs.
In 2018, Dr. Ruxin Li and his colleagues from Shanghai's Superintense Ultrafast Laser Facility started building a 100 PW (petawatt or 1015 Watts) pulsed laser known as the Station of Extreme Light (SEL).
By 2023, the SEL laser is expected to be fully operational, firing intense light pulses into a chamber 20 meters underground and subjecting targets to extreme temperatures and pressures.
The researchers plan to use the laser as a new way of accelerating particles and other high-energy physics experiments. But most fascinating use would be the demonstration that, at such extreme energy densities, light could directly create electrons and positrons from the vacuum.
Manipulating Antimatter with Lasers
Besides creating antimatter, physicists are also devising methods for manipulating and storing antiparticles and even entire antiatoms for long enough to be able to study their properties in detail.
Recently, researchers from the Antihydrogen Laser Physics Apparatus (ALPHA) collaboration at CERN demonstrated a successful manipulation of antimatter using a laser system that has the potential of transforming antimatter research.
Currently, a major limitation in antimatter experiments is that even magnetically trapped antihydrogen atoms still move randomly within the magnetic trap at velocities of up to 300 kilometers per hour. Much slower atoms are needed to facilitate future experiments where scientists aim to probe the gravitational free-fall of antihydrogen and to test the symmetry between matter and antimatter at an unprecedented level.
To slow down the antihydrogen atoms, the researchers at CERN utilized the fact that a beam of light can exert a mechanical force on both matter and antimatter without causing the latter to annihilate.
The mass of a single antihydrogen atom is so small (1.7 × 10–24 grams) that absorbing a photon of ultraviolet light (with a wavelength of 121.6 nm) imparts enough momentum to reduce the velocity of the antiatom by approximately 12 kilometers per hour. This effect is known as laser cooling and is widely used in ultra-low temperature experiments.
Laser Cooling Breakthrough for a Better Look at Antimatter
The ALPHA team applied laser cooling for the first time to antimatter by directing a train of relatively low-power laser pulses to a few dozen antihydrogen atoms, thus slowing them down to velocities below 50 kilometers per hour.
The antihydrogen atoms then spontaneously returned to their ground state by emitting another photon in a random direction. The whole process took several hours as each antiatom needed to absorb many photons to achieve substantial cooling. In future experiments, the researchers plan to increase the laser power and speed up the process.
Laser cooling opens exciting possibilities for studying antihydrogen. Combined with existing techniques that enable the accumulation of thousands of antihydrogen antiatoms per day, this novel approach could help scientists understand why matter is everywhere in our universe while antimatter is so elusive.
References and Further Reading
Hori, M. (2021) Antimatter cooled by laser light. Nature 592, 27-28. Available at: https://doi.org/10.1038/d41586-021-00786-6
Baker, C.J., et al. (2021) Laser cooling of antihydrogen atoms. Nature 592, 35–42. Available at: https://doi.org/10.1038/s41586-021-03289-6
B. Ham (2020) Making matter out of light: high-power laser simulations point the way [Online] Phys.org. Available at: https://phys.org/news/2020-05-high-power-laser-simulations.html (Accessed on 27 January 2022)
G. Sarri (2015) How we recreated the early universe in the laboratory [Online] The Conversation. Available at: https://theconversation.com/how-we-recreated-the-early-universe-in-the-laboratory-41399 (Accessed on 27 January 2022)
N. Madsen (2021) Antimatter: scientists find way to trap elusive material by blasting it with lasers [Online] The Conversation. Available at: https://theconversation.com/antimatter-scientists-find-way-to-trap-elusive-material-by-blasting-it-with-lasers-159307 (Accessed on 27 January 2022)
E. Cartlidge (2018) Physicists are planning to build lasers so powerful they could rip apart empty space [Online] Science News. Available at: https://www.science.org/content/article/physicists-are-planning-build-lasers-so-powerful-they-could-rip-apart-empty-space (Accessed on 27 January 2022)
Disclaimer: The views expressed here are those of the author expressed in their private capacity and do not necessarily represent the views of AZoM.com Limited T/A AZoNetwork the owner and operator of this website. This disclaimer forms part of the Terms and conditions of use of this website.