Since the advent of transistors, integrated circuits (ICs) have primarily relied on electrons for data transmission. These electronic ICs are supplemented by photonic building blocks such as lasers, waveguides, photodetectors, and optical fibers. However, internal interconnects and packaging are major challenges in traditional optical designs, limiting the scalability and integration of optical components in complex systems.
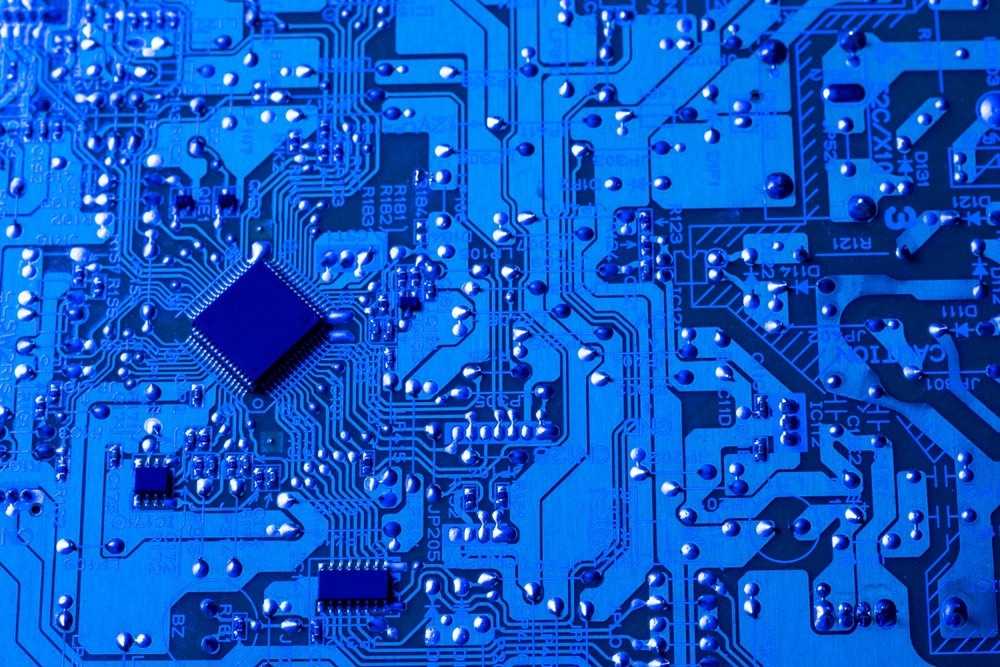
Image Credit: narong sutinkham/Shutterstock.com
Alternatively, photonic ICs (PICs) contain over 100 optical components on a single chip, offering greater functionality, reliability, and scalability. PICs represent the present and future technology for data centers and cloud computing, holding the potential to revolutionize telecommunications, computing, and medical diagnostics.1 This article explores the recent advancements, challenges, and prospects of PICs.
What are PICs?
PICs are advanced systems-on-a-chip that utilize optical carriers for high-speed data transmission. With the highly scaled integration of various optical components on micron to mm-size chips, they work in the visible and near-infrared (350-1650 nm) electromagnetic spectrum to perform complex functions like electronic ICs.
The basic components of PICs are modulators, switches, filters, attenuators, optical fibers, lenses, planar optical waveguides, photodetectors, light-emitting diodes, lasers, and optical amplifiers.1 These components are integrated on a single substrate through a top-down nanofabrication process that involves lithography, etching, and deposition systems installed in cleanroom facilities.2
The use of light for data transmission and processing in PICs offers multiple advantages over electronic ICs, including reduced size, weight, power consumption, and cost, along with higher bandwidth and speed.
PICs are aperture-independent due to fiber-coupling, transparent to modulation format, versatile, and scalable. PICs can overcome the limitations of electronic communication systems and discrete optical designs to provide a simpler, more reliable, and cost-effective solution for greater bandwidth communication.1
Recent Advancements in PIC Technology
A single, highly sophisticated, monolithic, Indium Phosphide (InP)-based PIC can contain over 1000 optical components.1 Recent advancements in membrane-based photonic technology could enable the integration of over 10,000 components per PIC. This further reduces size and energy requirements for higher-density integration and facilitates combination with electronic ICs.1
PICs can be fabricated from different materials, such as Silicon (Si), Silicon Nitride (SiN), Lithium Niobate (LiNbO3), and InP.2 The choice of substrate depends on the required functionality. For instance, silicon photonics is compatible with complementary metal-oxide-semiconductor (CMOS) technology, whereas InP is unique in offering direct integration of lasers.1,3
The type of optical components that can be integrated into a PIC also depends on the substrate material. For example, an InP-based PIC can accommodate lasers, modulators, amplifiers, and detectors, while a polymer PIC mainly consists of modulators and attenuators.1
Si and III-V semiconductors (InP, GaAs, InGaAs) form the backbone of communication technology.4 With the incorporation of small and pluggable transceivers, silicon photonics has the potential to support data transmission rates exceeding 100 Gb/s over distances of more than 10 km..1
Hybrid PICs have also been developed to overcome the limitations of individual materials. A silicon PIC with bonded InP lasers and amplifiers can reduce costs and maximize the scalability of silicon photonics.1
How are PICs Transforming Key Industries?
PICs are a next-generation disruptive technology with the potential to meet the power, size, weight, and performance goals of several sectors. These fields include telecommunications for faster internet, quantum computing for energy-efficient processors, healthcare for advanced diagnostic equipment, and aerospace for improved navigation systems.1,2
Another potential application of PICs is in modern gas sensing systems, with PIC-based optical spectroscopic sensors offering reductions in size and costs.5
The most prominent application of PICs is data communication, facilitated by the heterogeneous integration of on-chip laser sources. Si photonics-based light detection and ranging (LiDAR) systems have been developed for autonomous vehicles.
PICs have also been used in portable biosensors and lab-on-chip devices to monitor health vitals, including body temperature, glucose levels, heart rate, and blood pressure.3
Challenges and Prospects in PIC Evolution
Despite its potential to revolutionize various industries, PIC technology is not expected to achieve the same component density level observed in CMOS electronics, primarily due to constraints like optical component size, electrical connectivity, and thermal management.
The heat dissipation of photonic circuits is much larger than transistors, making resistive heaters a necessary component in PICs.1
Packaging constitutes over 60 % of the total production cost for PICs, and standardization in packaging processes remains elusive, leading to high variability in die and assembly processes.
The increasing demand for photonic-electronic integration (including electronic drivers, amplifiers, and control circuitry) complicates the packaging process further. Addressing these packaging challenges requires digitalization and the adoption of advanced techniques.1
Photonics research is also largely impacted by limited access to PIC fabrication facilities, the increased costs associated with PIC fabrication processes, and the prolonged device-to-design turnaround time.2 Most of the manufacturers and researchers work on an individual level, which makes processes like light source integration, fiber coupling, and alignment more tedious. Thus, significant upfront investments are required in PIC foundries to enable multi-project wafer runs.1
The integration platforms also suffer limitations like low optical power handling, high propagation losses, slow tuning response, and narrow transparency windows.1,3 no single substrate or technology can integrate the full range of photonic devices required in various applications on a single chip.1
Envisioning the Future of PICs
Programmable PICs are becoming increasingly recognized for their potential to develop flexible photonics for emerging technologies like optical computing, quantum computing, artificial intelligence, and neuromorphic photonics.2,3
These applications require the integration of tuneable components such as phase shifters, interferometers, and directional couplers into PICs. To rapidly realize such rewritable PICs at low costs, novel methods, such as direct laser writing on phase-change material thin films, are being explored.2
Integrated quantum photonics and quantum computing require compact PICs with high integration densities. Similarly, optical computing requires ultra-dense comb lasers for high-speed information processing with low latency and power consumption. The successful application of PICs in these areas requires the integration of on-chip light sources, achievable through advancements in nanofabrication techniques.3
Recent breakthroughs in material sciences, particularly with 2D materials (2DMs) such as graphene, black phosphorous, transition metal dichalcogenides, and ternary compounds, have opened new avenues for 2DM-PICs. These devices exhibit unique features like ultrafast carrier mobility, broadband absorption, strong nonlinear effects, and compatible monolithic integration.4
Other emerging PIC applications, such as flexible photonics, terahertz systems, microwave photonics, and mid-infrared photonics, require more advanced materials and nanofabrication processing.4 Integration of PICs with electronic ICs for drivers and logic in the future is expected to accelerate the adoption of PICs, potentially transforming technology and society.1
Through continuous research, investment, and collaboration, the current challenges facing PIC technology can be surmounted, unlocking its full potential across various sectors.
More from AZoOptics: Miniaturizing Quantum Devices with Novel Photonic Chips
References and Further Reading
1. Bozovich, A. (2020). Photonic Integrated Circuits (PICs) for Next Generation Space Applications. [Online] NASA. Available at: https://nepp.nasa.gov/docs/etw/2020/16-JUN-TUE/1345-Bozovich-NEPP%20ETW-CL20-2424-Photonics-v2.pdf
2. Wu, C., Deng, H., Huang, Y., Yu, H., Takeuchi, I., Rı́osC., Li, M. (2024). Freeform direct-write and rewritable photonic integrated circuits in phase-change thin films. Science Advances. doi.org/10.1126/sciadv.adk1361
3. Yang, J., Tang, M., Chen, S., Liu, H. (2023). From past to future: on-chip laser sources for photonic integrated circuits. Light-Science & Applications. doi.org/10.1038/s41377-022-01006-0
4. Wu, J., Ma, H., Yin, P., Ge, Y., Zhang, Y., Li, L., Zhang, H., Lin, H. (2021). Two‐Dimensional Materials for Integrated Photonics: Recent Advances and Future Challenges. Small Science. doi.org/10.1002/smsc.202000053
5. Hänsel, A., Heck, R. (2020). Opportunities for photonic integrated circuits in optical gas sensors. J Phys Photonics. doi.org/10.1088/2515-7647/ab6742
Disclaimer: The views expressed here are those of the author expressed in their private capacity and do not necessarily represent the views of AZoM.com Limited T/A AZoNetwork the owner and operator of this website. This disclaimer forms part of the Terms and conditions of use of this website.