Programmable integrated photonics offers reconfigurable on-chip platforms for implementing various photonic circuits through programmable elements, potentially leading to advancements in optical technologies, novel photonic functionalities, and deeper insights into light manipulation for various applications.
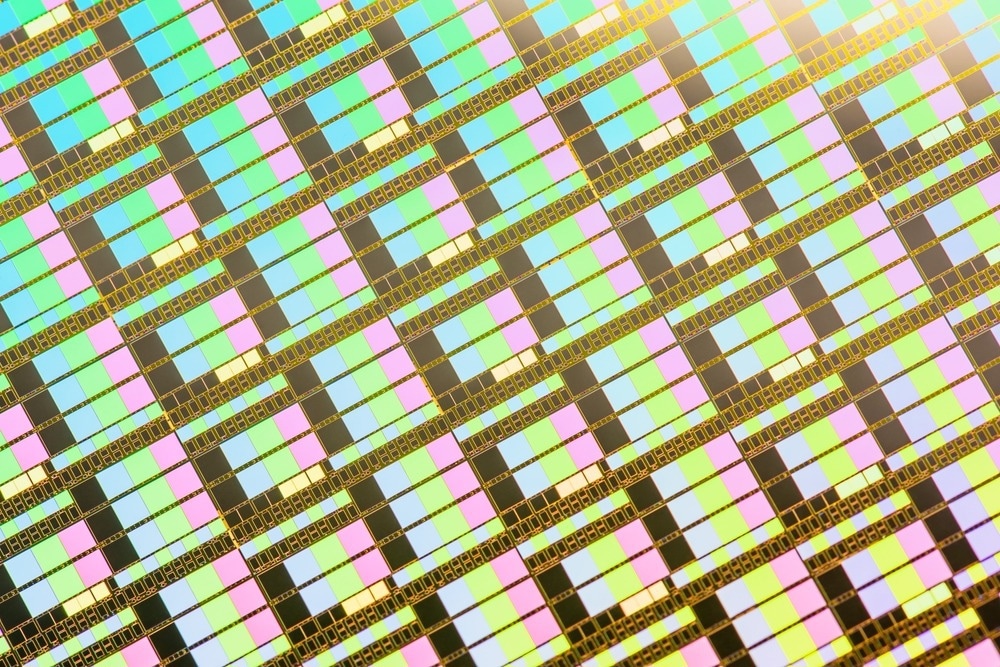
Image Credit: William Potter/Shutterstock.com
A recent study published in Nature Communications proposes and demonstrates a reprogrammable integrated photonics platform as a universal testbed for exploring various topological models, addressing limitations in existing photonic platforms designed for specific Hamiltonians with limited reconfigurability.
What is Programmable Integrated Photonics?
Programmable integrated photonics (PIP) deals with designing integrated optical hardware configurations that, through suitable programming and software, can execute a range of functionalities suitable for basic or complex operations in various application domains. This approach differs from the prevailing trend of application-specific photonic integrated circuits (ASPICs) that have dominated in recent decades.
PIP platforms typically consist of an interconnected mesh of basic photonic components with tunable parameters, such as phase shifters in Mach-Zehnder interferometers. By adjusting these parameters, light propagation through the circuit can be controlled dynamically via software to realize different functional modes. PIP thereby promises improved adaptability for multifunctional operation alongside benefits in scalability and cost-effectiveness by reusing the same hardware.
PIP's Role in Advancing Photonics Possibilities
An area poised to greatly benefit from the capabilities of PIP is topological photonics. Topological photonics introduces concepts of bulk boundary correspondence from condensed matter theory to create resilient edge modes in photonic materials with nontrivial topologies.
These modes have been demonstrated in various platforms, including 1D arrays of resonators or waveguides with chiral symmetries and 2D lattices of ring resonators and helical waveguides with asymmetric couplings.
While most topological photonics platforms are static, some reconfigurable topological photonic insulators have been experimentally realized in recent years, allowing for dynamic changes in guided wave pathways.
However, most of the existing topological photonic platforms can implement only one specific type of Hamiltonian with limited to no reconfigurability. They require extensive nanofabrication for building and have a fixed topological behavior once constructed. This restricts the exploration of different physics models and parameter spaces. Moreover, no reliable methods exist to systematically test device performance in case of disorder and fabrication variations.
Reconfigurable Photonic Platforms for Exploring 1D and 2D Topological Models
A recent study published in Nature Communications demonstrates how the versatility of PIP can overcome such constraints and provide a nearly universal testbed for topological photonics.
The researchers used a commercial programmable photonic platform, iPronics' Smartlight Processor, which featured a hexagonal mesh of silicon Mach-Zehnder interferometers with phase shifters.
In one demonstration, they specifically implemented a one-dimensional Su-Schrieffer-Heeger (SSH) Hamiltonian by configuring the mesh into a bipartite lattice of seven-ring resonators resembling the SSH model. This arrangement allowed them to demonstrate control over the band gap, localization degree, and topological edge mode robustness.
Another demonstration involved implementing a higher-order topological insulator based on a two-dimensional breathing Kagome Hamiltonian with three corner states. The researchers reconfigured the mesh into a 2D array of coupled ring resonators, which revealed corner-localized states in simulations, highlighting the platform's versatility.
This emphasizes that virtually any topological model could be implemented in programmable integrated photonic platforms, allowing for precise control over hopping strengths, phases, and onsite energies.
Significance of the Research
The study highlights the significant potential of employing programmable photonic meshes for manipulating light in topological configurations.
Promising directions include high-throughput testbeds for exploring novel topological physics, especially in areas like non-Hermitian, nonlinear, and synthetic dimensions.
The re-programmability facilitates accurate evaluations of robustness by introducing controlled defects, aiding in optimizing device performance for practical applications. Additionally, rapid design iterations become feasible, replacing time-intensive nanofabrication cycles with quick adjustments using programmable systems and on-chip monitors to optimize topological device prototypes.
While the findings are promising, there is ample room for innovation in enhancing the speed and precision of reconfigurability, particularly for investigating dynamic regimes. Integrating non-volatile tuning mechanisms could improve energy efficiency and allow for the retention of settings.
Incorporating on-chip characterization and metrology capabilities alongside reconfigurability could unlock autonomous optimization of topological order. In addition, machine learning integration may further aid in analyzing and improving topological performance metrics.
This research ushers programmable integrated nanophotonics into the topological realm, opening prospects for transformative photonic functionality. It presents a universal platform where myriad topological Hamiltonians across dimensions and symmetries become accessible within the same generalized co-processor implemented on standard PIP hardware.
By making topological photonic explorations more accessible in compact footprints without repeated nanofabrication, programmable integrated photonics promises to fast-track both fundamental topological physics studies alongside practical engineering of robust optical circuits and sensors.
More from AZoOptics: How do Optical Parametric Oscillators Work?
References and Further Reading
On, M. B., Ashtiani, F., Sanchez-Jacome, D., Perez-Lopez, D., Yoo, S. B., & Blanco-Redondo, A. (2024). Programmable integrated photonics for topological Hamiltonians. Nature Communications, 15(1), 629. https://doi.org/10.1038/s41467-024-44939-3
Capmany, J., & Pérez, D. (2020). Programmable integrated photonics. Oxford University Press. https://doi.org/10.1093/oso/9780198844402.001.000
Disclaimer: The views expressed here are those of the author expressed in their private capacity and do not necessarily represent the views of AZoM.com Limited T/A AZoNetwork the owner and operator of this website. This disclaimer forms part of the Terms and conditions of use of this website.