The Free Electron Laser (FEL) Prize is given to a person or people who have made a substantial contribution to the development of the free-electron laser field. The Award also offers the international FEL community the chance to honor one or more of its peers for their remarkable accomplishments.
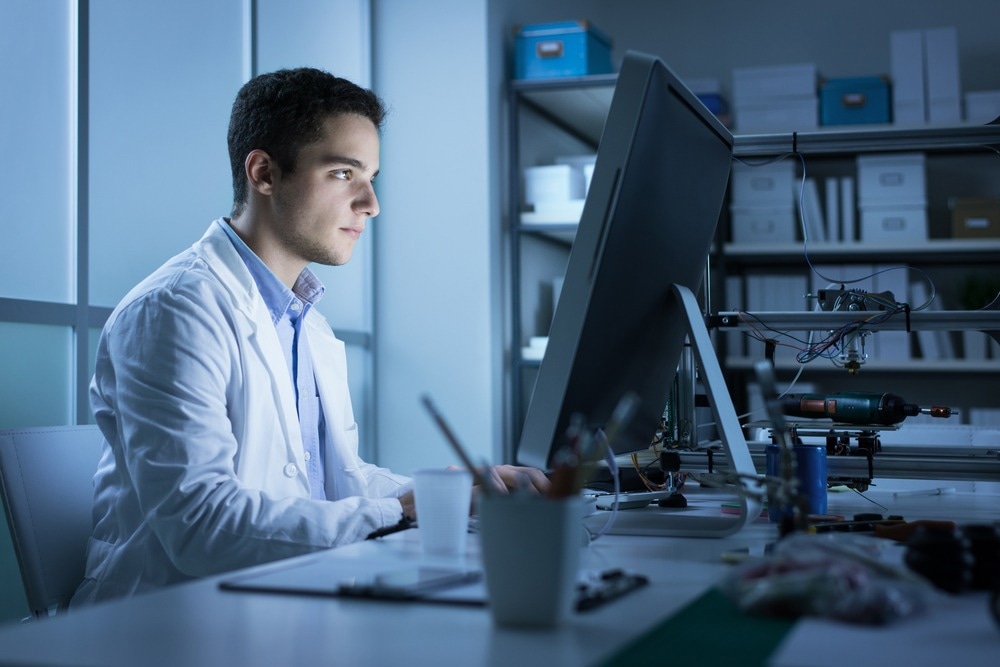
Image Credit: Stock-Asso/Shutterstock.com
In 1953, Hans Motz and his colleagues at Stanford University used the wiggler magnetic arrangement to create an undulator. An undulator consists of dipole magnets arranged in a periodic structure. Electrons moving through the periodic magnetic structure oscillate and release intense energy as a result. With this method, John Madey of Stanford University created the first free-electron laser in 1971. Madey amplified a signal using a 5 m long wiggler and a 43 MeV electron beam.
Working Principle of an FEL
A stream of electrons is accelerated close to the speed of light to produce an FEL. The electrons are directed along the wiggler along the longitudinal beam direction, while the direction across it is known as the transverse direction.
The electrons in the beam are forced to move transversely by the Lorentz force of the field and follow a sinusoidal pattern around the axis of the magnet array.
Because electromagnetic waves from randomly distributed electrons, they interfere both positively and negatively in time. When the electrons accelerate transversely, they produce monochromatic but incoherent photons. The radiation power increases linearly with the quantity of electrons. Standing waves of radiation are produced by the undulator's mirrors at either end or, alternatively, by an external excitation laser. When the radiation is powerful enough, the sinusoidal motion of the transverse electron current interacts with the transverse electric field of the radiation beam, causing some electrons to acquire energy and others to dissipate due to the ponderomotive force.
For a period of one optical wavelength, this energy modulation develops into variations in electron density. Hence, the electrons are longitudinally clumped into microbunches and separated along the axis by one optical wavelength. In-phase bunched electrons produced coherently combined radiation. In comparison, an undulator by itself generates electrons that radiate incoherently.
The electrons continue to microbunch as the radiation intensity increases. This process continues until a saturation power limit is reached that is much higher compared to the undulator.
By modifying the electron beam's energy or the magnetic field gradient of the undulators, the wavelength of the radiation emitted can be easily changed.
Advantages of FELs
The following are the main draws of free electron lasers:
- Their capacity to operate throughout a very wide wavelength range. Depending on the energy of the electrons, the FEL's wavelength can be anything from millimeters to x-rays.
- The wide wavelength tuning range can be achieved with a single device.
- High specifications on performance in wavelength ranges that are not accessible by conventional light sources.
The ability to produce high powers and their tunability make FELs highly attractive in many fields, including solid-state physics, surface studies, biophysical science, chemical technology, materials science, nonlinear spectroscopy, shock physics, solid density plasmas, and chemical engineering.
FEL Prize Winners
Along with the inventor of the FEL, John Madey, who received the first FEL Prize in 1988, 31 other prominent scientists have been honored with the Prize for their contributions. The full list can be found here. Some other notable recipients, the year of their award, and their achievements are noted below:
- Robert Phillips (1992) - For developing the Ubitron. The Ubitron is a high-power traveling-wave tube that interacts with a periodic electron beam that has been magnetically undulated.
- Claudio Pellegrini (1999) - For his innovative work on X-ray free electron lasers (XFEL) and relativistic particle beams and collective effects.
- Li-Hua Yu (2003) - The self-amplified spontaneous emission free electron laser (SASE FEL) and the high gain harmonic generation free electron laser (HGHG FEL) were developed by Yu and colleagues.
- Avraham Gover (2005) - For creating the innovative theoretical groundwork for the FEL hypothesis.
- Vladimir Litvinenko and Hiroyuki Hama (2004) - A number of FELs were constructed by Litvinenko and his team using the optical klystron, a more sophisticated FEL design. The group was the first in the world to extend a FEL's range to ultraviolet wavelengths in 1988 and to vacuum ultraviolet in 1999, respectively.
- Zhirong Huang and William Fawley (2014) - Jeong created the first millimeter-wave FEL followed by the first compact terahertz FEL. Fawley was awarded the FEL Prize for his work in creating early FEL simulation algorithms.
- Bruce Carlsten, Dinh Nguyen and Richard Sheffield (2017) - For their innovative work on the first operational RF photo-injector and the first theoretical model that introduced the idea of emittance correction.
Future Outlook
Given the high annual publishing rate and the diverse and dynamic character of the papers involved, it is clear that the science, technology, and applications of FELs have led to significant breakthroughs on all fronts.
It is crucial to highlight that there is now a greater demand for X-ray FEL access globally due to the rapid growth of FEL science and technology. The governments of advanced economies recognize that investing in national facilities is crucial to maintaining competitiveness on the international stage. National facilities not only contribute to scientific advancement but also to the development of competitive advantage for the national industrial base, key technology development, and skill development.
More from AZoOptics: What is Photoacoustic Spectroscopy?
References and Further Reading
E A Seddon et al, Short-wavelength free-electron laser sources and science: a review. 2017 Rep. Prog. Phys. 80 115901. DOI 10.1088/1361-6633/aa7cca
P. G. O’Shea, H. P. Freund, Free-electron lasers: Status and applications. Science 292, 1853–1858 (2001).
McNeil, B., Thompson, N. X-ray free-electron lasers. Nature Photon 4, 814–821 (2010). https://doi.org/10.1038/nphoton.2010.239
Disclaimer: The views expressed here are those of the author expressed in their private capacity and do not necessarily represent the views of AZoM.com Limited T/A AZoNetwork the owner and operator of this website. This disclaimer forms part of the Terms and conditions of use of this website.