Medical diagnostic methods based on light have become a reliable tool over the last 50 years. Lasers, in general, offer a non-invasive method to test infections and alterations in biological samples.
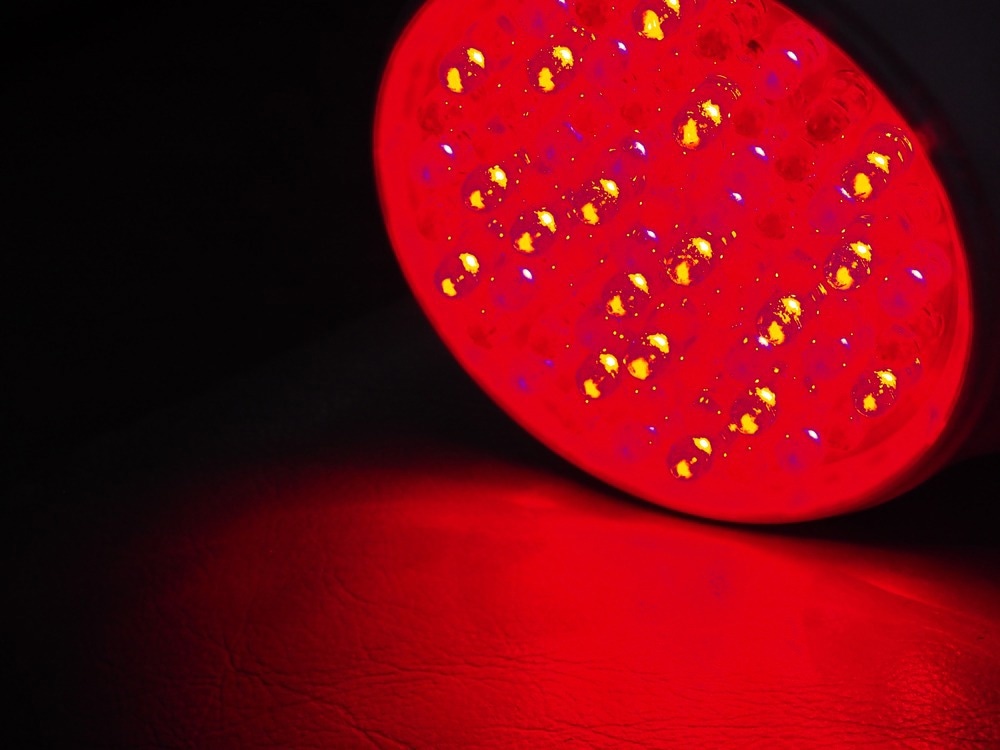
Image Credit: Pamela Carpenter/Shutterstock.com
Continued research and development in the optical diagnostic field is working towards improving the laser source, the components used in delivering the light to the sample, and also on detection and analysis.
Infrared (IR) light is especially useful in molecular analysis. This is because the internal structure of molecules actively interacts with different portions of the IR spectrum. For example, vibrational and rotational transitions in molecules are excited by IR light.
Development of Optical Sources
Many research efforts have helped develop laser sources that radiate in the IR spectrum. The properties of such systems have to be tailored to match the application for which they are used. Lasers are characterized by their lasing modes and associated features. For example, lasers can emit in continuous wave (CW) or pulsed mode. Pulsed lasers are capable of delivering high-energy bursts of photons. Such pulses can drive molecular effects that are critical for analyzing biological matter.
Laser pulses are described by their pulse widths and repetition rates. The pulse width of a laser is the time elapsed between the rising and falling edges of a single pulse. For example, the rising point and the falling point of a sinusoidal wave along an axis.
Repetition rate is the count of how many pulses are emitted in one second. The repetition rate is reported in frequency units. Lasers are developed at various pulse widths and repetition rates to address different applications.
An advantage of precisely controlling the pulse features of the laser oscillation is the use of its nonlinear processes. Understanding and controlling how the waveform of light changes over time can be used to study electronic interactions at sub-femtosecond timescales.
Most materials used for producing laser emission in the IR spectral region work in the near IR (NIR) part of the spectrum. While many experiments are conducted in the NIR spectral region, laser sources in the mid-IR also have numerous applications. Examples of applications other than medical diagnostics include electron orbital imaging, spectroscopy of semiconductor nanostructure, and low-frequency photonics, among many others.
Nonlinear Frequency Generation
Nonlinear frequency generation (NLFG) is exploited to achieve laser emission in the mid-IR.
NLFG overcomes the lack of direct laser emitting media in the mid-IR. NLFG processes utilize nonlinear crystals that have the inherent property to absorb light at a particular frequency and emit it at a new shifted frequency.
For example, in second harmonic generation (SHG), a light incident at 1064 nm on a nonlinear crystal is emitted at 532 nm after NLFG.
Mid-IR wavelengths produced by NLFG only span an octave or more of the fundamental wavelength. This limitation produces discrete segments of wavelengths in the mid-IR.
Continuous phase control across the entire mid-IR spectrum has not yet been achieved. Not having continuous access to the vast mid-IR bandwidth curtails the extent of possible diagnostic implementations.
Adaptive and beam conditioning optics used with NLFG widen the wavelength coverage. But the complexity of implementing such an instrumental setup has been challenging. Even when properly executed, the reproducibility of the output waveforms has been difficult to maintain until recently.
Infrared Waveform Control
A research collaboration between physicists from the Ludwig Maximilian University of Munich (LMU), the Max Planck Institute of Quantum Optics (MPQ), and the Hungarian Center for Molecular Fingerprinting (CMF) have demonstrated precisely controlled single-cycle waveforms in ultrashort mid-infrared pulses.
The new technique developed emits mid-IR radiation using multi-octave NLFG.
The emerging waveforms have the unique capability to change the relative phase at multiple spectral regions.
Cascaded intrapulse difference-frequency generation (IPDFG) is used to produce mid-IR waveforms in different spectral bands. The spectral phases are then manipulated by exploiting the electric and optical characteristics of the driving pulse. The output waveforms are continuously adjustable and the evolution of the electric field is highly reproducible.
Outlook
Having access to a broad IR range of controlled pulsed light increases the ability to analyze the composition of molecules more precisely.
Identifying unique features allows for diagnosing diseases such as cancer. For example, a developing tumor slightly alters the molecular composition of the blood. IR analysis can quickly detect anomalies and enable early detection.
Pulse-controlled IR waveforms offer significant upgrades to reliable optical imaging. IR optical imaging has been established as a powerful approach to studying the metabolic dynamics of lipids and proteins.
The IPDFG-based approach has the potential to detect previously undetectable variations in particular biomolecules. The degree of light pulse control and mid-IR wavelength coverage afforded by the new method increases the reliability of future medical diagnostics.
References and Further Reading
Steinleitner, P., Nagl, N., Kowalczyk, M. et al. Single-cycle infrared waveform control. Nat. Photon. (2022). https://doi.org/10.1038/s41566-022-01001-2
Optics.org (June 06, 2022). Munich partnership controls light pulses in MIR range | Research & Development. https://optics.org/news/13/6/9
Pupeza, I., Huber, M., Trubetskov, M. et al. Field-resolved infrared spectroscopy of biological systems. Nature 577, 52–59 (2020). https://doi.org/10.1038/s41586-019-1850-7
Disclaimer: The views expressed here are those of the author expressed in their private capacity and do not necessarily represent the views of AZoM.com Limited T/A AZoNetwork the owner and operator of this website. This disclaimer forms part of the Terms and conditions of use of this website.