Article updated on 4 May 2022
Electron microscopy has opened new worlds of detail in materials science, eclipsing the capabilities of conventional light microscopes with much higher resolution. Various types of electron microscopes have been pioneered to address different needs in high-resolution imaging applications. The two mainstay techniques are transmission electron microscopy (TEM) and scanning electron microscopy (SEM). Both methods use an electron source to create an image of a sample's surface or its inner structure, with the distinct advantage of achieving sub-angstrom spatial resolution. The capabilities of both TEM and SEM imaging are such that atomic-level information is now routinely accessible.1
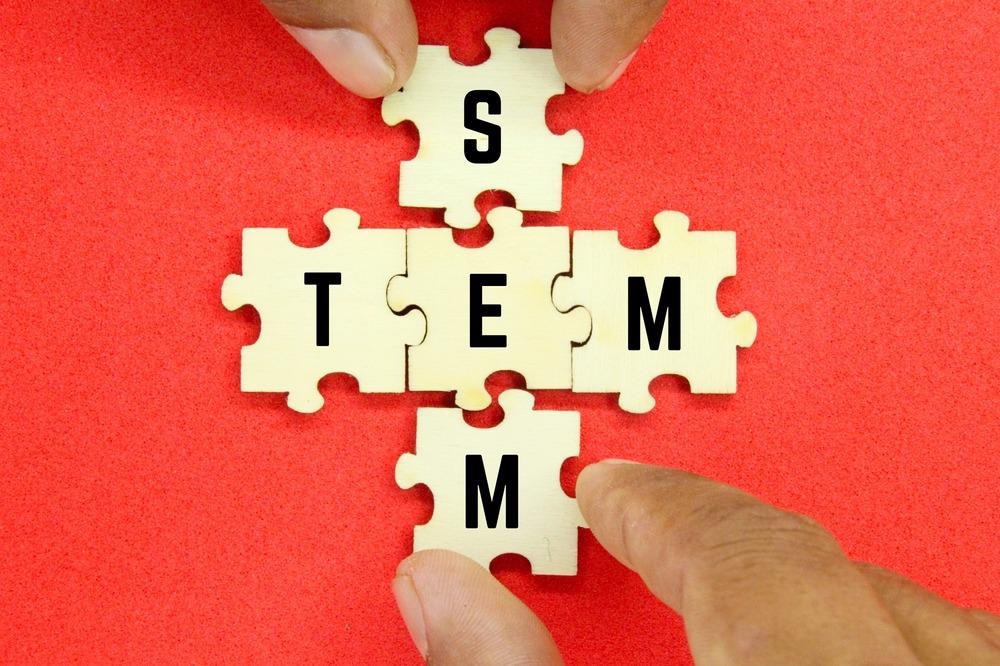
Image Credit: Fauzi Muda/Shutterstock.com
The main advantage of electron microscopy over light microscopy is the limited achievable resolution that comes with using visible light. The diffraction limit for visible light means that the best spatial resolution that can be achieved is approximately ~ 200 nm. While super-resolution methods can now surpass this, many of these methods require the use of specific fluorophore labels which limits their generality. Electron microscopy does not require staining, marking SEM and TEM among the most attractive imaging platforms in modern science. 2
This article will briefly outline the advantages of TEM and SEM systems, while addressing the difference between scanning electron microscopes and transmission electron microscopes.
The Working Principles of Scanning Electron Microscopy
Scanning electron microscopes - often initialized to SEM, or even SEM microscopes - work by using an electron gun to generate a beam of electrons. These are then tightly focused using stacks of electron lenses. As all electrons have the same negative charge, one of the big challenges with maintaining focused beams in electron microscopy is handling the space charge repulsion. Brighter electron beams with a large number of electrons allow for better illumination of the sample but space charge effects also start to become more significant.
Once the electron beam has been generated and focused, it passes through the column of the instrument onto the sample. The sample is mounted onto a stage and the resulting backscattered and secondary electrons are detected. Some microscopes can also detect the X-ray fluorescence generated during the electron interaction with the sample. As the energies of the detected X-rays are element-specific, these can be used to build up a profile of the elemental composition of the sample.
The electron beam can be rastered over the surface of the sample to achieve larger scanning areas and build up a more complete picture of the sample of interest.
The challenge in SEM sample preparation is removing contaminants that may interfere with the imaging process as well as ensuring there is no water present as under the high vacuum conditions this will also obscure the regions of interest. Samples do have to be relatively durable for use in SEM as they must be able to withstand the high vacuum conditions used.
Key Applications of SEM
Materials characterization has been one huge area of application for SEM. This includes more traditional metallic materials such as gold surfaces but also soft, organic materials such as polymers.
SEM is being used to identify how different blends of biopolymers interact and behave with a view to identifying which compositions might be beneficial for the development of new, biodegradable materials.3
The high spatial resolution of SEM makes it a popular tool in the manufacturing of a variety of materials and for quality control, particularly for the manufacturing of highly regulated devices such as those for healthcare.4 SEM can be used to identify any surface defects and check for the presence of any elemental contaminants if the microscope has the ability to detect X-rays.
Another advantage of this high spatial resolution is for applications in microelectronics and industries that rely on the use of microscopic components. For the cosmetics industry, where many products are formulated as particles, SEM can be used to check the typical particle sizes as well as to characterize their surface characteristics. This can ensure that all the synthesized particles meet the same uniformity tolerance standards and that the final product will have the expected properties.
How Transmission Electron Microscopy Differs from SEM
Another alternative, or sometimes complementary, electron microscopy to SEM is transmission electron microscopy (TEM). Transmission electron microscopes operate on many of the same principles as their scanning electron microscopy equivalents.
TEM instruments make use of bright electron beams generated by an electron gun that are then focused onto the sample. The key difference between the two techniques is that in an SEM experiment, once the sample has been irradiated, the electron emitted from the sample is detected. In TEM, the electron beam passes straight through the analyte of interest, and the change in the transmission is instead recorded.
Key Applications of SEM
TEM is a very powerful tool for examining the structure of solid materials and can also be used with tissue samples.5
It has even been used to identify antigens in biomedical samples to diagnose the presence of certain bacteria as it can identify characteristic features on cell walls. It is a popular technique for highly crystalline structures, particularly for nanotechnology applications where high spatial resolution is essential.
Differences Between SEM Vs TEM
While both methods use the same electron generation and control methods, sample preparation and requirements for TEM are very different. TEM requires thin layers of samples (usually < 150 nm) for sufficient amounts of electrons to be transmitted. While that means it is sensitive to what is inside the sample, TEM recovers flatter, 2D-like images, whereas SEM can be used for full reconstructions.
The advantage of TEM over SEM is the further enhanced image resolution. By obtaining transmitted electrons, TEM images recover more information on the internal structure of the object, unlike SEM where only the scattered electrons as used. However, the acquisition of secondary and backscattered electrons following raster scanning of a surface provides unparalleled surface and compositional detail, making SEM a powerhouse in surface imaging.
SEM Vs TEM: Choosing the Right System
Different applications require different tools. SEMs provide in-depth information on sample surfaces, enabling users to acquire detailed 3D images. TEMs, meanwhile, offer 2D projections of a sample's internal structure. Choosing scanning between SEM and TEM primarily depends on the type of analysis you plan to conduct.
References and Further Reading
- Smith, D. J. (2008). Ultimate resolution in the electron microscope? Materials Today, 11, 30–38. https://doi.org/10.1016/S1369-7021(09)70005-7
- Huang, B. (2010). Super-resolution optical microscopy: multiple choices. Current Opinion in Chemical Biology, 14(1), 10–14. https://doi.org/10.1016/j.cbpa.2009.10.013
- Rydz, J., Šišková, A., & Andicsová Eckstein, A. (2019). Scanning Electron Microscopy and Atomic Force Microscopy: Topographic and Dynamical Surface Studies of Blends, Composites, and Hybrid Functional Materials for Sustainable Future. Advances in Materials Science and Engineering, 2019. https://doi.org/10.1155/2019/6871785
- Goldstein, J. I., Newbury, D. E., Michael, J. R., Ritchie, N. W., Scott, J. H. J., & Joy, D. C. (2017). Scanning electron microscopy and X-ray microanalysis. Springer.
- Arenal, F. L., A., D., & R., M. (2015). Advanced transmission electron microscopy. Springer
Disclaimer: The views expressed here are those of the author expressed in their private capacity and do not necessarily represent the views of AZoM.com Limited T/A AZoNetwork the owner and operator of this website. This disclaimer forms part of the Terms and conditions of use of this website.