An interview with Dr Juan Sebastian Totero Gongora and Prof. Marco Peccianti, from the Emergent Photonics (EPic) Lab at the University of Sussex conducted by Alina Shrourou, BSc.
Please give an overview of how and why cameras are used at Terahertz (THz) frequencies?
Terahertz (THz) is a quite unexplored part of the electromagnetic spectrum which lies between the microwave (used for example in satellite communications) and the visible light. The main reason behind this inaccessibility is that the required technology has not been available until the 80s-90s and became reasonably affordable even much later.
Terahertz waves are characterised by a much larger wavelength (slightly below one millimetre) than visible light (corresponding roughly to less than one-millionth of a mm). As such, THz waves can easily penetrate common materials (e.g., paper, clothes, plastic) and THz technologies are being widely developed in the context of security scanning and manufacture control, as they allow to see inside objects and wrapping.
At the same time, however, materials react quite differently to THz light. Biological samples and complex molecules, for example, respond with a characteristic electromagnetic signature when shined with THz radiation, allowing researchers to discriminate materials which are almost indistinguishable with visible light. This possibility has enormous potential in developing critical applications, such as explosives detection, medical diagnostics, and food safety.
Available Terahertz cameras are still a relatively primitive device, mostly quite insensitive and expensive to fabricate. However, single pixel Terahertz detectors are currently more advanced in the sense that they allow perceiving how the terahertz waves are made in their time evolution, revealing the spectroscopic signature of the desired object (e.g. detecting an explosive). Perhaps the most advanced and accessible form of Terahertz camera is a single detector that scans an object moving in front of it.
What are the limitations of current Terahertz imaging methods?
THz radiation is extremely hard to detect, and it requires expensive technologies to build the THz equivalent of optical cameras. When it comes to retrieving the exact nature of the terahertz, all the current approaches either require unreasonable powers, or they very slow and so are of very limited practical importance. The challenges increase in identifying small objects, as needed for example in many biomedical applications. With THz waves, this is exceptionally challenging, due to the substantial difference in scale between THz wavelengths and the typical dimensions of the samples of interest.
The capability of observing an object depends on the ratio between its size and the illuminating wavelength. As a rule of thumb, objects which are smaller than the wavelength are very hard to image with standard techniques. Such constraint represents a considerable limitation as many of the primary objects of interest (e.g., biological cells or molecules) are several orders of magnitude smaller the THz wavelength.
What is Nonlinear Ghost Imaging?
To overcome these limitations, we decided to follow an entirely different approach. Instead of considering a camera with a sensor composed of many separate pixels (as in a standard commercial camera), we propose a new type of device consisting of just a single pixel, which captures all the THz light transmitted or reflected by the object.
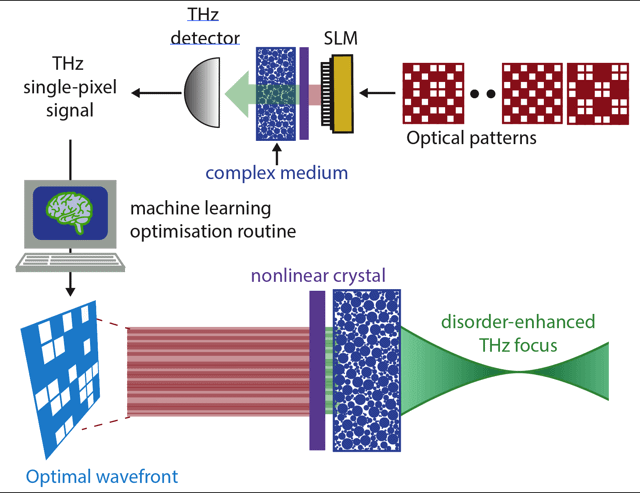
Single pixel THz imaging can be applied to engineer advanced functionalities in complex photonics media.
By using this simple single-pixel sensor, we have shown how we can reconstruct the object at very high resolution by illuminating it with a rapid sequence of different THz-wave patterns.
The idea of employing single-pixel cameras and THz patterns for imaging has been discussed in the last few years by several top-level research groups. Previously, all approaches imitated a popular optical methodology (the Ghost Imaging) which has been extensively developed in the visible and infrared spectrum.
The Nonlinear Ghost Imaging is inspired by the latter, with the key difference that the THz-wave patterns are generated from optical pattern through a conversion process which is, in physical description, defined as “Nonlinear”. Because of this conversion, the Nonlinear Ghost Imaging is founded on different physics than the Ghost Imaging (for example, the latter does not contain any spectral information of the object).
Please outline your recent research where you discovered this unique imaging concept.
Our specific innovation is that we show how the Nonlinear Ghost Imaging simultaneously achieves high resolution and obtains spectral information at THz of the object. More generally, our recent work theoretically demonstrates that this approach represents the "proper" way to understand what the object is made of with great accuracy.
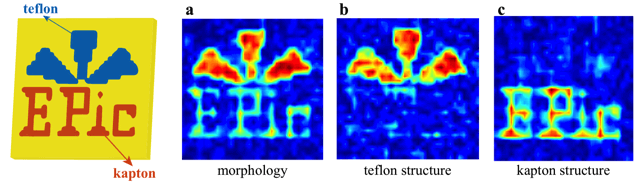
Using time-resolved nonlinear ghost imaging it is possible to retrieve the material composition of an object.
Please explain how the conventional limitations of Terahertz imaging could be negated with Nonlinear Ghost Imaging.
To acquire the total information of an object in the THz domain, we need to make an image in space and time. This allows us to see a "movie" of what happens to the object when a pulse travels in it.
The analogous optical approach operates only in space, so you can just see the average light that passes through the object. This approach simply cannot work to extract complete information at THz, although, at low resolutions, an unknown error (distortion) in the image might be considered acceptable. We understood where this error lies, and we outlined the correct approach to extract the image from those patterns. The Nonlinear Ghost Imaging is an embodiment of this general approach.
How is possible that a single pixel camera that uses this technique can not only image the shape of an object, but it can also determine its chemical composition?
In light of the innovation of our approach, we can record in time how the properties of a THz pulse, a brief emission of radiation, changes as it passes through the object and produces an image of the object “in-time” which reveals its nature (i.e. the material it is composed of). Compared to the prior art in single pixel images, we have demonstrated not only that in our methodology the resolution is inherently higher but that we can also discriminate different materials.
What do you see as the key application areas that will benefit from the use of Nonlinear Ghost Imaging?
Conceptually it applies in all those fields where distortion-free images and spectroscopic information of an object are required, with a rapid imaging time. Security, such as body scanners, and microscopy appear to be potential targets.
Could this technique be adapted for other imaging systems, or is it limited to Terahertz imaging?
All these features represent a considerable improvement when compared to established technologies, and they have a huge potential impact beyond the field of THz cameras. More in general, in fact, this technique could be employed to design high-resolution cameras in other frequency ranges different from visible light, such as infrared and microwaves.
These devices would be extremely interesting for applications as, for example, they could be employed to develop collision sensors and ultra-rapid radars for self-driving cars or a novel generation of body scanners. Ultrasound imaging could also benefit from this specific advancement. While at this stage our work is mostly theoretical and based on simulations, in our laboratory we are currently working on experimentally demonstrating our device.
What’s next in your research, and how do you plan on taking this from a mostly theoretical standpoint to a functional imaging technique?
This project is funded by the European Research Council (Horizon 2020 - GA n° 725046. for a value of about 2M Euro), and this achievement represents only one of its initial deliverables. The project target is to produce a prototype operating using state-of-the-art high energy lasers sources (the experimental setting is already in deployment at the Emergent Photonics Lab). It is important to highlight that the field lacks an established solution with the capabilities proposed here.
The development of this device would be critical in part of our current investigation on complex photonic systems, which constitute one of our core research interests. Complex photonic systems, such as random distributions of particles or corrugated surfaces, are composed of a large number of interacting components. When the number of interactions becomes extremely large, these systems can spontaneously develop advanced functionalities, in analogy with how intelligence and consciousness emerge from the interaction of billions of neurons in the brain. Single-pixel imaging can be effectively employed to characterise and engineer the optical properties of these systems to develop optical devices, such as high-resolution lenses or optical neural networks.
Where can readers find more information?
All the information about the progress of this project can be found on the laboratory news http://www.sussex.ac.uk/physics/epic/ or more specifically in the project-hub.
About Dr. Juan Sebastian Totero Gongora
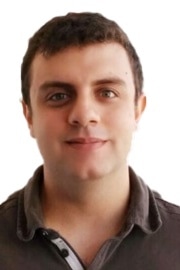
Juan Sebastian Totero Gongora is a Research Fellow in Experimental Photonics at the University of Sussex, United Kingdom. After graduating from Sapienza University, Italy, he obtained his Ph.D. in electrical engineering at KAUST, Saudi Arabia, where his research focused on light–matter interactions in complex metamaterials, disorder-enhanced optical systems, and integrated nanolasers.
Despite his young age, his publications include 14 journal publications, two book chapters, 34 conference contributions and one international patent on early-stage cancer detectors. His current research focuses on the theoretical and experimental investigation ultrafast nonlinear photonics, nonlinear THz imaging and photonic neuromorphic computing.
About Prof. Marco Peccianti

Marco Peccianti s Full Professor at the University of Sussex (UK) and co-director of the Emergent Photonics Lab. He is editor for the Optical Society of America, chair and member of several scientific committees, and author of about 105 journal publications, 210 conferences, book chapters and patents (with more than 7000 citations).
He is a recipient of the EU ERC-Consolidator grant TIMING and of several personal prizes and fellowships. He also investigator in the UK Quantum Technology Hub for Sensor and Metrology. His current research interests include the experimental investigation of terahertz physics and ultrafast nonlinear optics.
Disclaimer: The views expressed here are those of the interviewee and do not necessarily represent the views of AZoM.com Limited (T/A) AZoNetwork, the owner and operator of this website. This disclaimer forms part of the Terms and Conditions of use of this website.