EPFL scientists have established a hybrid device that considerably progresses existing, ubiquitous laser technology.
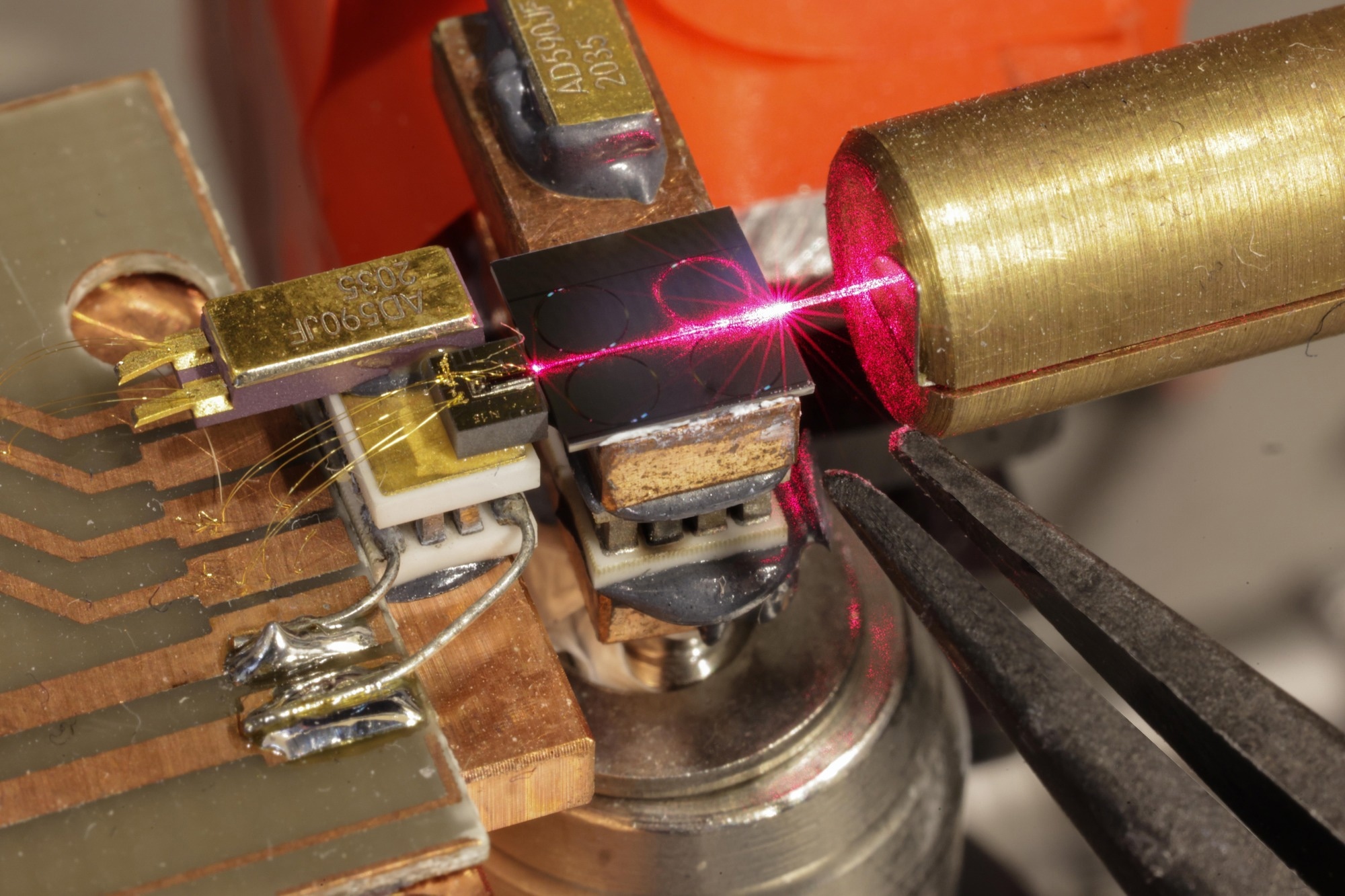
The micro-resonator being activated by a semi-conductor laser. Image Credit: 2023 EPFL/Alain Herzog - CC-BY-SA 4.0
By creating a chip-scale laser source, the researchers at EPFL's Photonic Systems Laboratory (PHOSL) have improved semiconductor laser performance and made it possible to produce shorter wavelengths.
With implications for metrology, telecommunications, and other high-precision applications, this groundbreaking discovery, coordinated by Professor Camille Brès and Postdoctoral Researcher Marco Clementi from EPFL's School of Engineering, represents a substantial advance in the field of photonics.
The study describes how the PHOSL researchers, working with the Laboratory of Photonics and Quantum Measurements, successfully integrated semiconductor lasers with silicon nitride photonic circuits that contained microresonators.
It was published in the journal Light: Science & Applications. After this integration, the industry is faced with a hybrid device that closes a long-standing technological gap by producing light that is very uniform and precise in both the visible and near-infrared regions.
Semiconductor lasers are ubiquitous in modern technology, found in everything from smartphones to fiber optic communications. However, their potential has been limited by a lack of coherence and the inability to generate visible light efficiently. Our work not only improves the coherence of these lasers but also shifts their output towards the visible spectrum, opening up new avenues for their use.
Camille-Sophie Brès, Professor, Photonic Systems Laboratory (PHOSL), École polytechnique fédérale de Lausanne
In this context, coherence relates to the consistency of the phases among the emitted light waves from the laser. Elevated coherence implies synchronization among these waves, resulting in a highly precise beam characterized by specific color or frequency.
This attribute holds immense importance in applications demanding exactness and steadiness in the laser beam, notably in fields like timekeeping and precise sensing.
Increased Accuracy and Improved Functionality
The team's strategy revolves around integrating off-the-shelf semiconductor lasers with a silicon nitride chip fashioned using widely adopted, cost-effective CMOS technology. Leveraging the material's remarkable low-loss characteristics, minimal light absorption or leakage occurs.
Laser light travels through minute waveguides on the chip into minuscule cavities, known as micro-ring resonators, where it becomes confined. These intricately crafted cavities are precisely engineered to resonate at particular frequencies, amplifying desired wavelengths selectively while dampening others. This meticulous process culminates in heightened coherence within the emitted light.
Another notable feat lies in the hybrid system's capacity to twofold the frequency of light emitted by the commercial semiconductor laser—effectively transitioning from the near-infrared spectrum to the visible light spectrum.
The correlation between frequency and wavelength dictates an inverse relationship; doubling the frequency entails halving the wavelength. While near-infrared wavelengths find application in telecommunications, higher frequencies hold significance in crafting compact, efficient devices requiring shorter wavelengths, as observed in atomic clocks and medical instruments.
The achievement of shorter wavelengths occurs through a process called all-optical poling, which occurs as the confined light within the cavity triggers second-order nonlinearity within the silicon nitride. In this context, nonlinearity denotes a substantial, disproportionate change in the light's behavior, not directly proportional to its frequency, due to its interaction with the material.
Ordinarily, silicon nitride doesn't exhibit this specific second-order nonlinear effect, prompting the team to engineer a sophisticated solution: Leveraging the light's resonance within the cavity, the system generates an electromagnetic wave that induces these nonlinear properties within the material.
An Enabling Technology for Future Applications
We are not just improving existing technology but also pushing the boundaries of what's possible with semiconductor lasers. By bridging the gap between telecom and visible wavelengths, we’re opening the door to new applications in fields like biomedical imaging and precision timekeeping.
Marco Clementi, Postdoctoral Researcher, Photonic Systems Laboratory (PHOSL), École polytechnique fédérale de Lausanne
Metrology, especially the creation of small atomic clocks, is one of this technology’s most promising uses. The capacity to carry precise timepieces has been essential to the development of navigation throughout history, from measuring longitude at sea in the 16th century to guaranteeing precise navigation during space missions and improving geo-localization in the present day.
Clementi notes, “This significant advancement lays the groundwork for future technologies, some of which are yet to be conceived.”
The team’s extensive knowledge of material science and photonics may result in lighter, smaller devices as well as cheaper, more energy-efficient laser production. Their capacity to take a basic scientific idea and turn it into a useful application through industry-standard fabrication highlights the possibility of resolving difficult technical problems that may result in unanticipated advancements.
Journal Reference
Clementi, M., et al. (2023). A chip-scale second-harmonic source via self-injection-locked all-optical poling. Light: Science & Applications. doi/s41377-023-01329-6