In order to better understand quantum physics, advance quantum information processing, and create quantum technologies, photons have served as a flagship system. The utilization of photons as a naturally mobile, low-noise system with widely available quantum-limited detection led to the demonstration of quantum entanglement, teleportation, quantum key distribution, and early quantum computer demonstrations.
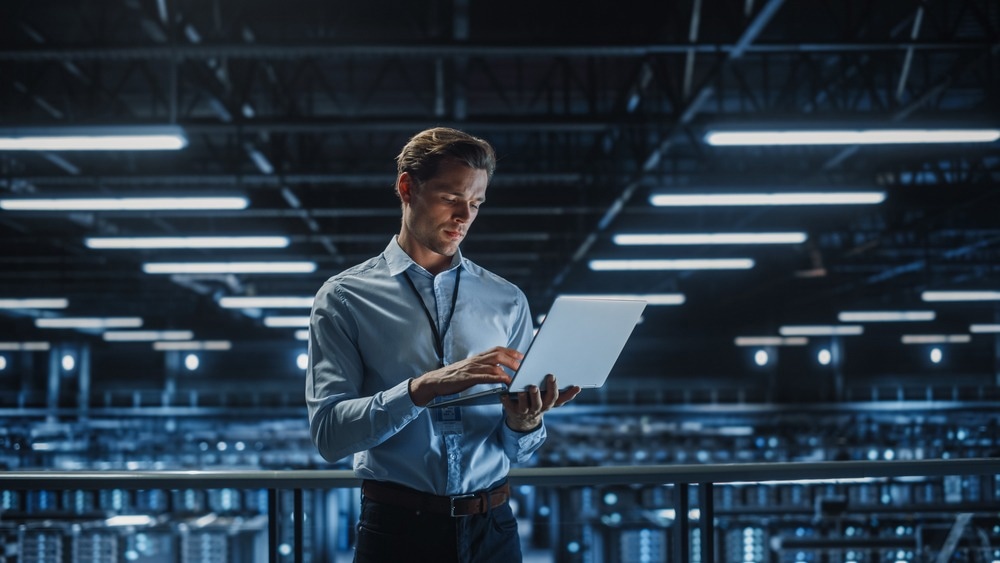
Image Credit: Gorodenkoff/Shutterstock.com
Mathematical issues that can't be resolved by ordinary computers are anticipated to be resolved by quantum computers. Particularly in the fields of cryptography, secure communications, pharmacological research, logistics, etc., many of these issues are practically significant.
Superposition, entanglement, and interference are examples of natural phenomena that quantum computers use to their advantage. Quantum bits (qubits) are used to process information that is represented by certain quantum states. One option for the physical foundation of the qubits needed for quantum computation is a quantum system, which can be created using atoms, electrons, ions, or light-based techniques like photons.
Photonic Qubits
The photonic technique offers many advantages, including infinite coherence time, information processing at room temperature, flying qubits beneficial for platform interconnection, and an established semiconductor industry.
Due to their lack of mass and electric charge, photons are light particles that have unlimited coherence time and are less vulnerable to environmental disturbances. Encoding information in these is an effective technique to securely transfer data because single photons can also be produced in a range of configurations.
The ability to simultaneously generate a large number of single photon states is necessary for performing computing activities in the short and long term. The obvious method to do this is to have a lot of deterministic sources, each of which is capable of concurrently producing one and only one photon upon the occurrence of a trigger event.
These photons also need to be:
- Efficiently collected to enter the PQC processor and avoid loss due to absorption, diffraction, scattering, or mode mismatch.
- In a pure quantum state and identical to one another
- Has to be detected efficiently and be compatible with low-loss materials used in detection technology.
Various physical systems, including laser cooled atoms, trapped ions, defects in materials, quantum dots, and other more advanced techniques, are being used to create genuinely deterministic, high-quality photon sources like this. While some of them rely on the employment of a single emitter, which in theory inherently enables on-demand single-photon emission, others—like atomic ensemble and parametric nonlinear processes—need heralding signals and switching to make them work.
Generating Photons
Spontaneous parametric downconversion (SPDC), a crucial technique for experimental quantum optics, continues to be a workable method for producing high-quality single photons in a nondeterministic manner. The features listed above have been successfully handled by developments in this technology.
Although SPDC is probabilistic, it can be used to create "heralded" single photon states, in which the detection of a photon's twin serves as a sign that one is there. In contrast, SPDC can generate photon pairs that are intrinsically entangled in frequency, transverse spatial modes, or polarization.
Encoding Qubits
Probability amplitudes related to the photon occupation of two modes with a certain degree of freedom in the optical field can be used to encode qubits. This method is referred to as Dual-rail encoding. A simple explanation can be provided using waveguides.
Inside a waveguide, photons travel in a single path. Two modes and one photon can encode one qubit. Using two waveguides as modes, each mode's unit of light is used to represent a qubit. The photon can occupy one of the modes. A photon on an arbitrary position, upper waveguide can correspond to a qubit in state |0> and a photon on the lower waveguide to a qubit in state |1>.
Performing Quantum Computations
Using this qubit platform, computations must be performed. To do so, some fundamental components and stages have to be developed, much as each step is a gate on a classical computer.
For instance, to initialize quantum superposition where the photon is situated 50% of the time in the higher waveguide and 50% of the time in the lower waveguide, beam splitters are employed. A beam splitter is an optical instrument that takes two inputs and delivers two outputs. The solitary photon will randomly migrate to either of the modes with a 50/50 chance after it enters a beam splitter. Additionally, various probabilities, such as 30-70% or 40-60%, may be required; in that case, more advanced beam splitters incorporating phase shifters are required.
Detection
In a quantum experiment, a photon's life begins with generation and ends with detection. Both processes must be effective, and PQC depends heavily on their performance and qualities.
Every moment a photon strikes an ideal photon detector (PD), it clicks and promptly resumes operation. In cases where no actual photons were found, it does not generate misleading positive signals. Additionally, it specifies the number of photons that were found in the same spatiotemporal mode.
Such perfect photon detectors are not yet available. Accordingly, the detection efficiency, reset time, detection time jitter, dark count rate, and photon-number-resolving capacities of existing PDs are continuously advanced.
Although a "perfect" PD is not absolutely necessary for PQC, a realistic and scalable platform depends on enhancing the PD performance to very high levels.
Future Outlook
Modern electro-optic components, such as integrated electro-optic modulators or Pockels cells, enable quick polarization switching to perform demanding Bell tests with the locality and freedom of choice in closed or spatial mode switching for source multiplexing purposes. The development of effective tools for manipulating more unusual degrees of freedom, like frequency-time or transverse spatial modes, is also ongoing. These tools include methods for converting information from one degree of freedom to another, like polarization to spatial transverse mode, discrete variable to continuous variable, frequency conversion, and others.
In the long run, there is a lot of promise. The overhead and error thresholds are being reduced thanks to advancements in cluster-state methods created expressly for photonics, especially for loss. Along with large-scale integrated platforms, exceptionally high-quality sources, detectors, and gates are enabling the hardware advancements needed to create processors with a large number of components. It is possible to demonstrate a true quantum computing advantage sooner rather than later by using intermediate tasks like Boson Sampling. For remote entanglement sharing in general, as well as for tying together processors that are geographically separated, photonics remains the leading platform.
Advancements in Fiber Optic Communication: From Single-Mode to Multi-Core Fibers
References and Further Reading
Sergei Slussarenko, Geoff J. Pryde; Photonic quantum information processing: A concise review. Appl. Phys. Rev. 1 December 2019; 6 (4): 041303. https://doi.org/10.1063/1.5115814
T. G. Tiecke, J. D. Thompson, N. P. de Leon, L. R. Liu, V. Vuletić, and M. D. Lukin, “Nanophotonic quantum phase switch with a single atom,” Nature 508, 241 (2014).https://doi.org/10.1038/nature13188
Quandela Team. (31 March 2022) How to do computations on an optical quantum computer?. [Online] Quandela. Available at: https://medium.com/quandela/how-to-do-computations-on-an-optical-quantum-computer-a0c579bebeb0
Disclaimer: The views expressed here are those of the author expressed in their private capacity and do not necessarily represent the views of AZoM.com Limited T/A AZoNetwork the owner and operator of this website. This disclaimer forms part of the Terms and conditions of use of this website.