Fluorescence Lifetime Imaging Microscopy (FLIM) has emerged as a transformative tool for unraveling intricate molecular interactions. By harnessing the unique ability of fluorophores to reveal dynamic processes, FLIM unveils hidden complexities within cellular environments, offering unprecedented insights into the fundamental molecular interactions that underlie biological phenomena. This article offers a comprehensive overview of FLIM and its applications at the molecular level.
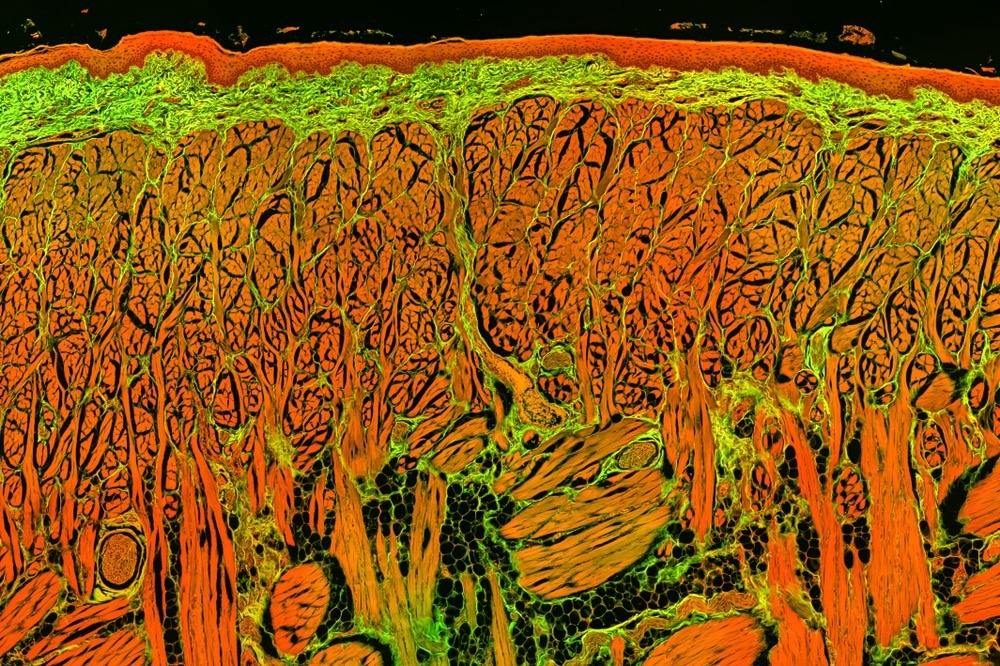
Image Credit: Micha Weber/Shutterstock.com
What is Fluorescence Lifetime Imaging Microscopy (FLIM)?
Fluorescence lifetime imaging microscopy (FLIM) is an advanced imaging technique that leverages the fluorescence lifetime of fluorophores to provide unique insights into molecular dynamics and interactions. The fluorescence lifetime refers to the average duration a fluorescent molecule remains excited before emitting a photon and returning to the ground state.
Its independence from factors like concentration, absorption, sample thickness, and photobleaching enhances its robustness, while its sensitivity to environmental parameters such as ion concentration, pH, molecular binding, and energy acceptor proximity makes it ideal for functional imaging.
FLIM's capabilities extend to studying protein-protein or DNA-protein molecular interactions using Förster resonance energy transfer (FRET) sensors, contributing to our understanding of cellular signaling and molecular interactions.
Despite past complexity, recent technological advancements have made FLIM label-free, faster, and more accessible, rendering it suitable for studying cellular signaling and molecular interactions.
How to Impliment FLIM
There are two primary implementations of FLIM:
- Time-Domain FLIM: In this method, the sample is stimulated with a short pulse, and the decay process is assessed either by tracking photon arrival times grouped into a histogram or through techniques involving pulse sampling or time-gated detection. In cases of multiple fluorescent species, their contributions are combined into a unified histogram.
- Frequency-Domain FLIM: Each photon is assigned a phase delay relative to the excited wave, similar to an arrival time histogram. In the case of multiple fluorescent species, this phase distribution is subjected to Fourier analysis to extract parameters for demodulation and modulation, facilitating species separation.
Both frequency and time-domain FLIM have distinct advantages and challenges. They cater to different FLIM scenarios, such as high dynamic range imaging, low photon budget imaging, and high time resolution imaging.
Advantages of FLIM Over Traditional Intensity Imaging
While intensity imaging shows fluorophore distribution and can be differentiated by spectral properties, FLIM excels at distinguishing molecular environments or similar spectra around the same fluorophore. For example, FLIM easily distinguishes Nicotinamide adenine dinucleotide phosphate (NAD(P)H) in various cellular contexts, which is challenging with intensity-based methods.
FLIM is insensitive to fluorophore concentration changes, enabling differentiation between changes in quantum yield and overall concentration. It's less affected by inner filter effects, making it ideal for precise quenching dynamics measurement.
FLIM is self-referenced, not needing throughput calibration, making it reproducible across setups. In addition, it's unaffected by scattering and can account for scattering profiles using fitting routines, ensuring accuracy even in deeper penetration.
Investigating Molecular Interactions Using FLIM
Oxygen Imaging
FLIM enables the measurement of oxygen concentrations within cells through oxygen-quenching, a process that reduces fluorescence lifetime.
This technique is particularly sensitive when applied to fluorophores with extended fluorescence lifetimes, such as long-lived ruthenium dyes (t>800 ms). These dyes serve as effective oxygen reporters in FLIM-based oxygen imaging, requiring specialized FLIM equipment that operates at lower pulse repetition rates or very low modulation frequencies (kHz range).
Enhanced Calcium Monitoring in Brain Signaling through FLIM
Calcium ions (Ca2+) are essential for brain signaling, and Oregon Green BAPTA-1 (OGB-1) dye's high sensitivity to nanomolar [Ca2+] through fluorescence lifetime enables accurate mapping of resting [Ca2+] in neurons and astroglia.
In a study published in Neuron, Zheng's team developed an advanced two-photon excitation time-resolved imaging method that uses the high sensitivity of OGB-1 fluorescence lifetime to correlate nanomolar Ca2+ concentrations. This technique allows them to acquire data rapidly and provides accurate [Ca2+] readouts unaffected by various factors.
Compared to intensity-based approaches, the proposed FLIM-based overcomes the limitations of intensity-based measurements and provides a high-resolution mapping of basal [Ca2+] in neurons and astrocytes.
The study highlights OGB-1's resilience to micro-viscosity, temperature, pH fluctuations, and Mg2+ and Zn2+, establishing it as a valuable lifetime-based sensor for tracking resting [Ca2+] in brain slices and in vivo.
Characterizing Molecular Dynamics of Single Dye Molecule with Confocal FLIM
A study published in Single Molecules focuses on confocal fluorescence lifetime imaging microscopy (Confocal FLIM) of single dye molecules immobilized on a glass surface. The researchers employed a short-pulse diode laser emitting at 635 nm to investigate the time-resolved identification of specific oxazine and carbocyanine dyes based on their specific fluorescence lifetimes.
They observed fluctuations in fluorescence lifetime and intensity of individual molecules (at millisecond resolution), attributed to transitions between different quantum states, including the triplet state, and correlated with rotational and spectral jumps.
This work introduces a novel approach to monitoring and understanding dynamic processes at the molecular level, offering valuable insights into the behavior of individual dye molecules and their interactions on surfaces.
Protein-Protein Interactions
FRET-FLIM is an advanced imaging technique combining FLIM with Förster Resonance Energy Transfer (FRET) to study cell molecular interactions. Its application in studying the epidermal growth factor receptor (EGFR) has provided details on receptor oligomerization and activation.
FRET-FLIM techniques have unveiled pre-dimerized receptors, assessed EGFR phosphorylation status, and visualized receptor activation propagation in living cells. In addition, advanced FRET-FLIM methods, including rFLIM and emFRET, have also contributed to ErB1-signaling research.
Beyond membrane signaling, FRET-FLIM has illuminated downstream cytosolic targets, revealing molecular events in protein kinase C (PKC)-signaling, transcription factor interactions, and disease-related protein interactions such as those involving Rab-GTPases, amyloid precursor protein, and tetanus toxin. This technique has proven instrumental in elucidating intricate protein interactions across diverse cellular processes.
Simultaneous Multi-RNA Imaging in Cells Using Advanced FLIM Technique
RNA dynamics in live cells offer valuable biological insights, often achieved by genetically fusing fluorescent aptamer tags to target RNAs. While efforts have enhanced features such as RNA-probe binding affinity, there is a significant interest in improving multiplexing for simultaneous visualization of multiple RNA species.
In a study published in Nature Communications, the researchers developed a fluorescence lifetime-based RNA tagging platform called Riboglow-FLIM to enhance accuracy and enable the simultaneous visualization of multiple RNAs.
By employing smaller RNA tags derived from a bacterial riboswitch sequence family, Riboglow-FLIM offers better contrast and versatility.
This approach enabled the researchers to visualize two different RNAs in live cells simultaneously, a challenge not achieved by previous methods.
Future Outlooks
FLIM has revolutionized our understanding of molecular interactions, enabling dynamic insights into cellular environments and medical advances. With advancing technology and interdisciplinary collaborations, FLIM's future promises transformative discoveries, providing unprecedented clarity into the intricacies of biological systems.
More from AZoOptics: Time-Lapse Microscopy in Cell Biology
References and Further Reading
Datta, R., Heaster, T. M., Sharick, J. T., Gillette, A. A., & Skala, M. C. (2020). Fluorescence lifetime imaging microscopy: fundamentals and advances in instrumentation, analysis, and applications. Journal of biomedical optics, 25(7), 071203-071203. https://doi.org/10.1117/1.JBO.25.7.071203
Dr. Lioba Kuschel, James DeRose, Leica Microsystems. (2022). What is FLIM - Fluorescence Lifetime Imaging Microscopy? [Online]. Available at: https://www.leica-microsystems.com/science-lab/what-is-flim-fluorescence-lifetime-imaging/
PicoQuant. (2023). Fluorescence Lifetime Imaging (FLIM)-An imaging technique that uses fluorescence lifetime of fluorophores to generate additional contrast. [Online]. Available at: https://www.picoquant.com/applications/category/life-science/fluorescence-lifetime-imaging-flim
Sarfraz, N., Moscoso, E., Oertel, T., Lee, H. J., Ranjit, S., & Braselmann, E. (2023). Visualizing orthogonal RNAs simultaneously in live mammalian cells by fluorescence lifetime imaging microscopy (FLIM). Nature Communications, 14(1), 867. https://doi.org/10.1038/s41467-023-36531-y
Tinnefeld, P., Buschmann, V., Herten, D. P., Han, K. T., & Sauer, M. (2000). Confocal fluorescence lifetime imaging microscopy (FLIM) at the single molecule level. Single Molecules, 1(3), 215-223. https://doi.org/10.1002/1438-5171(200009)1:3%3C215::AID-SIMO215%3E3.0.CO;2-S
van Munster, E. B., & Gadella, T. W. (2005). Fluorescence lifetime imaging microscopy (FLIM). Microscopy Techniques: -/-, 143-175. https://doi.org/10.1007/b102213
Zheng, K., Bard, L., Reynolds, J. P., King, C., Jensen, T. P., Gourine, A. V., & Rusakov, D. A. (2015). Time-resolved imaging reveals heterogeneous landscapes of nanomolar Ca2+ in neurons and astroglia. Neuron, 88(2), 277-288. https://doi.org/10.1016/j.neuron.2015.09.043
Disclaimer: The views expressed here are those of the author expressed in their private capacity and do not necessarily represent the views of AZoM.com Limited T/A AZoNetwork the owner and operator of this website. This disclaimer forms part of the Terms and conditions of use of this website.