Optical MEMS technology integrates mechanical elements, electronics, and sensors on a silicon substrate through microfabrication, enabling unprecedented miniaturization and integration in optical systems. It has become a driver for the commercial development of optical systems and is a focus of extensive research into the fundamentals of optical interactions.
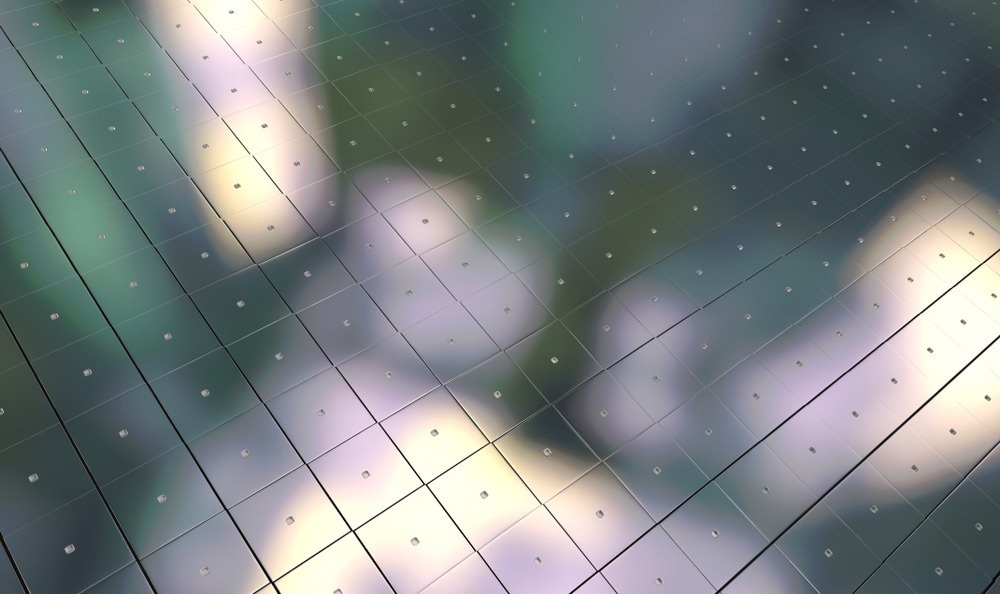
Image Credit: Peter Sobolev/Shutterstock.com
What is Optical Micro-Electro-Mechanical Systems (MEMS) Technology?
Optical micro-electro-mechanical systems (MEMS) combine electrical, mechanical, and optical systems to detect and manipulate optical signals at the micron level. It leverages batch fabrication techniques from the semiconductor industry to achieve miniaturization, extraordinary precision, and reliability.
Optical MEMS has achieved enormous commercial success in displays, projectors, and fiber optic communications. In addition, it has enabled the miniaturization of devices and various micromechanical elements, leading to cost-saving batch production in the same way as integrated circuits.
Optical MEMS devices can control and modify optical parameters such as beam direction and focal length, enabling the development of miniaturized tunable mirrors, lenses, modulators, and filters.
These devices can be easily scaled up to high-volume production despite the initial effort and time required for the development. In addition, micro/nanofabrication techniques adopted from the semiconductor industry allow for higher precision in micro-optical systems.
Optical MEMS is a relatively new and rapidly advancing technology that has the potential to revolutionize photonic systems and transform telecommunications, optical networking, and biomedical applications by enabling the development of a new family of miniaturized devices.
Design and Fabrication of Optical MEMS Devices
Fabricating optical MEMS devices involves microfabrication techniques like bulk and surface micromachining and deep X-ray lithography.
The fabrication consists of functionality testing of optical and electronic structures, followed by developing and analyzing mechanical elements. The final step involves depositing materials, etching, and creating patterns.
The design of optical MEMS devices depends on various parameters such as shape, stress distribution, electrical, mechanical, chemical, stiffness, material, and thermal parameters for proper functioning.
Applications of Optical MEMS Devices
Optical MEMS plays a significant role in telecommunications, particularly in developing all-optical switches for routing internet traffic through fiber optic networks. The demand for faster internet speeds has pushed the development of optical MEMS systems, which can directly manipulate optical signals and eliminate the need for unnecessary conversions.
Optical MEMS technology is extensively used in projection, transmissive, and reflective displays. These displays can be categorized as scanning-based and non-scanning-based, further dividing the former into linear array 1D and point source 2D scanning-based displays.
It enables the realization of small-sized spectroscopic systems such as grating-based monochromators, Fourier transform spectrometers, and Fabry–Perot interferometers. In addition, the use of MEMS components also allows for the development of portable spectroscopy systems.
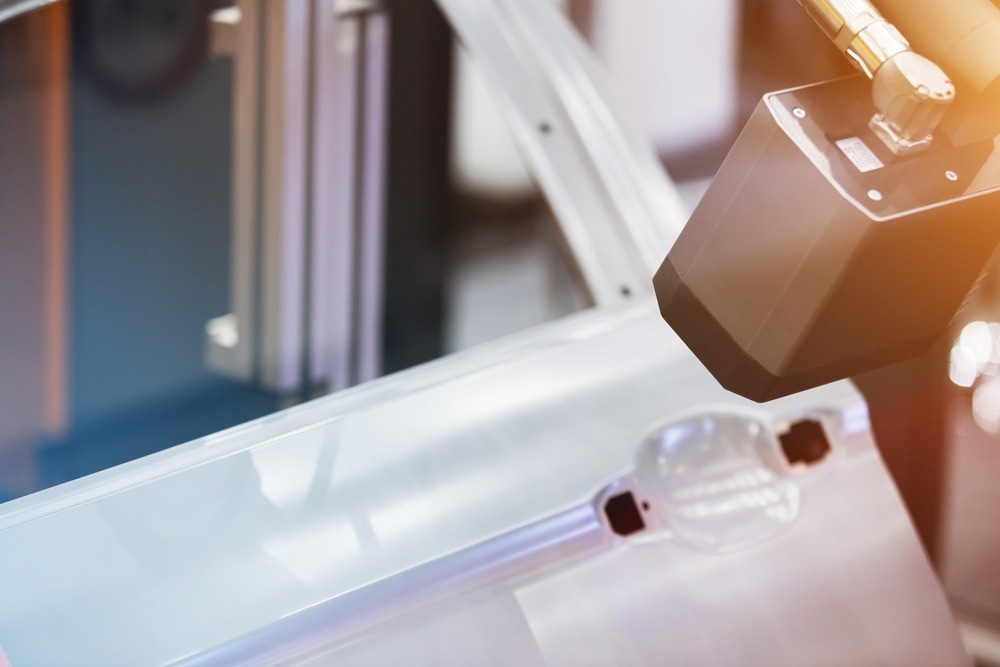
Image Credit: MOLPIX/Shutterstock.com
Optical MEMS technology is used in sensors, which can be categorized as coherent and noncoherent. Coherent sensors rely on light interference, while noncoherent sensors detect variations in light intensity.
Optical MEMS devices have enabled numerous applications in the biomedical field, such as optogenetics, molecule detection, and noninvasive endoscopic screening for diseases. In addition, miniaturizing biological tools through Bio-MEMS devices has enabled real-time monitoring and manipulation of biological processes.
Recent Research and Development
Real-Time Vibration Sensing with Highly Sensitive Optical MEMS Accelerometer for Vehicles and Seismic Applications
A study published in Applied Optics presented the development, production, and testing of an optical MEMS accelerometer that uses the Talbot effect of double-layer diffraction gratings for highly sensitive acceleration detection.
The researchers optimized the grating parameters using finite-difference time-domain simulation to achieve optimal contrast for optical interferometric detection.
Experimental results demonstrate that the proposed optical MEMS accelerometer design achieved high resolution (246 µg), sensitivity (6.1 V/g), and bias stability (0.02 mg) and can operate at higher accelerations (∼80g).
The study's significance lies in its potential application in real-time geophysical seismic and vibration sensing in vehicles, where fast and strong vibrations require accurate detection.
Optical MEMS-based Smart Glass Technology Revolutionizes Energy Efficiency in Buildings
In a study published in the Journal of Optical Microsystems, researchers from the University of Kassel in Germany demonstrated a new smart glass technology using optical MEMS micromirror arrays in smart windows for daylight steering, which can provide huge energy savings.
The designed MEMS micromirror array is installed inside insulated glazing, enabling light customization directly by reflecting incoming sunlight according to user preferences, time of day, sun location, and season.
The smart glazing provides free overheating prevention in summer, solar heating in winter, healthy natural daylight, up to 35% energy savings, up to 30% carbon dioxide reduction, and a 10% reduction in steel and concrete in high-rise buildings.
This technology can reduce reliance on artificial lighting and optimize natural daylight, reacting to local climate and saving substantial energy year-round.
Researchers Develop an Advanced Silicon Optical MEMS Platform for Large-Scale Photonic Integrated Circuits
Researchers led by Associate Professor Niels Quack from the University of Sydney developed a silicon optical MEMS platform that fully integrates high-performance nano-opto-electromechanical devices with silicon photonics foundry components. The results are published in Microsystems & Nanoengineering.
This technology overcomes the challenges of integrating many components on a single chip. It allows creating large-scale photonic integrated circuits for various applications, including telecommunications, sensing, and quantum computing.
This is the first time that nano-electro-mechanical actuators have been integrated in a standard silicon photonics technology platform. It is an important step towards mature large-scale, reliable photonic circuits with integrated MEMS. This technology is being prepared for high-volume production, with potential applications in 3D imaging for autonomous vehicles or new photonic assisted computing.
Niels Quack, Associate Professor, Lead Author of the Study.
Future Outlooks
The high demand for system miniaturization has led to a billion-dollar global market for MEMS and optical MEMS, with many products for daily life.
With the progress in nanofabrication technology, further scaling MEMS down to Nanoelectromechanical systems (NEMS) is now possible. Moreover, the fusion of NEMS/MEMS with nanophotonic elements will create new opportunities and functional photonic devices and systems with enhanced performance, dynamic tunability, and a higher level of integration.
More from AZoOptics: Realization of Photonic Metamaterial-Based Continuous Time Crystal
References and Further Reading
Hillmer, H., Iskhandar, M. S. Q., Hasan, M. K., Akhundzada, S., Al-Qargholi, B., & Tatzel, A. (2021). MOEMS micromirror arrays in smart windows for daylight steering. Journal of Optical Microsystems, 1(1), 014502-014502. https://doi.org/10.1117/1.JOM.1.1.014502
Quack, N., Takabayashi, A. Y., Sattari, H., Edinger, P., Jo, G., Bleiker, S. J., ... & Bogaerts, W. (2023). Integrated silicon photonic MEMS. Microsystems & Nanoengineering, 9(1), 27. https://doi.org/10.1038/s41378-023-00498-z
Jin, L., Wang, C., Jin, L., Chen, W., Xu, H., Cui, M., & Li, M. (2022). Micro-opto-electro-mechanical systems accelerometer based on the Talbot effect of double-layer diffraction gratings. Applied Optics, 61(18), 5386-5391. https://doi.org/10.1364/AO.457479
Solgaard, O., Godil, A. A., Howe, R. T., Lee, L. P., Peter, Y. A., & Zappe, H. (2014). Optical MEMS: From micromirrors to complex systems. Journal of Microelectromechanical Systems, 23(3), 517-538. https://doi.org/10.1109/JMEMS.2014.2319266
Upadhyaya, A. M., Hasan, M. K., Abdel-Khalek, S., Hassan, R., Srivastava, M. C., Sharan, P., ... & Vo, N. (2021). A comprehensive review on the optical micro-electromechanical sensors for the biomedical application. Frontiers in Public Health, 9, 759032. https://doi.org/10.3389/fpubh.2021.759032
Grüger, H. (2021). MOEMS and MEMS‐Technology, Benefits & Uses. Portable Spectroscopy and Spectrometry, 89-113. https://doi.org/10.1002/9781119636489.ch5
Motamedi, M. E. (2005). MOEMS: Micro-opto-electro-mechanical Systems (Vol. 126). SPIE press. https://doi.org/10.1117/3.2265061
Zhou, G., & Lee, C. (Eds.). (2017). Optical MEMS, Nanophotonics, and their applications. CRC Press. https://www.optica-opn.org/home/book_reviews/2018/0818/optical_mems_nanophotonics_and_their_application/
Jiang, H. (2016). Optical MEMS for chemical analysis and biomedicine (No. 137791). IET. Available at: https://digital.library.tu.ac.th/tu_dc/frontend/Info/item/dc:137791
Piyawattanametha, W., & Qiu, Z. (2012). Optical MEMS. InTech. https://doi.org/10.5772/2761
Disclaimer: The views expressed here are those of the author expressed in their private capacity and do not necessarily represent the views of AZoM.com Limited T/A AZoNetwork the owner and operator of this website. This disclaimer forms part of the Terms and conditions of use of this website.