Understanding the relationship of the atoms' vibrational patterns at the level of chemical bonding is invaluable for the advancement of material science. A new collaborative study between Vanderbilt University and the University of the Chinese Academy of Sciences has observed the vibrational patterns in the conducting materials silicon and graphene, which is a breakthrough in the field.
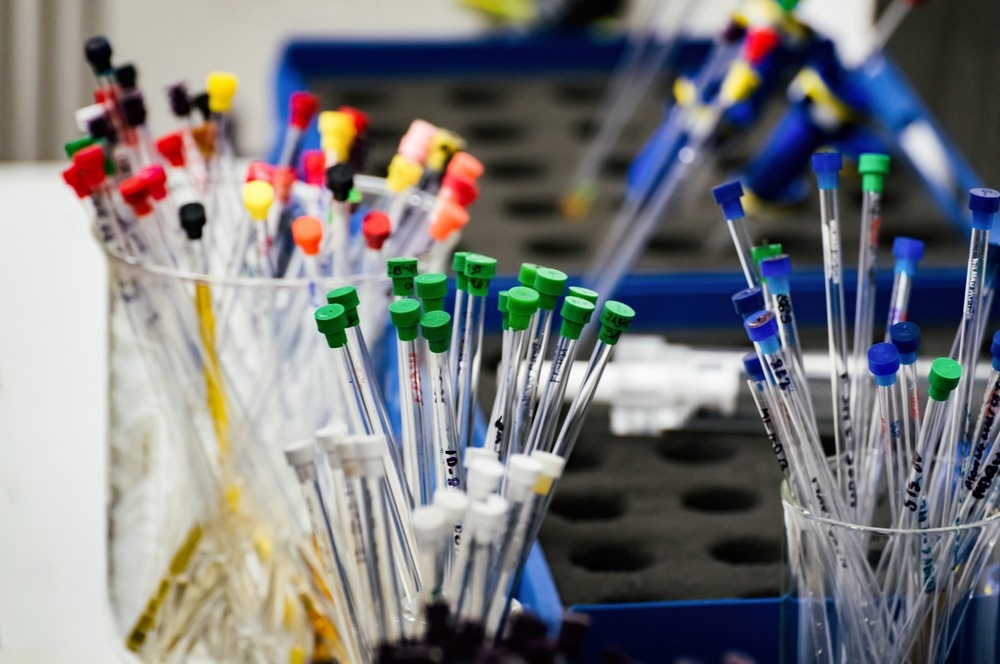
Image Credit: Forance/Shutterstock.com
It is crucial to understand atomic vibrational patterns because, among other things, they determine a material's capacity to transfer heat. The functioning of the semiconductors in computers and smartphones depends on proper heat management. Nanophotonics, a branch of nanotechnology, also requires careful heat control. Nanophotonics, sometimes known as nano-optics, is a subfield of photonics that focuses on understanding how light behaves at the nanometer scale and how nanometer-sized objects interact with light.
The current study successfully pushed the chemical-bonding-configuration limit of single-atom vibrational spectroscopy sensitivity. The process of measuring the vibrational patterns of individual atoms near silicon impurities in graphene, shown by the researchers, has the potential to completely alter how heat-conducting materials and structures that power electronics and batteries are designed. Graphene, also called the "wonder material," is now better understood thanks to new, accurate studies of its vibrational characteristics, which has significant ramifications for the usage of graphene in anything from supercomputers to nanoantennas for chemical and biological sensing.
The researchers showed how the atomic mass of impurity atoms and chemical-bonding configurations affect local vibrational characteristics at the single-atom level. They did this by combining experimental and theoretical methods.
Scanning Transmission Electron Microscope (STEM) and Electron Energy-loss Spectroscopy (EELS)
The experimental techniques employed in this research included scanning transmission electron microscope (STEM) and electron energy-loss spectroscopy (EELS) measurements. The research team put in a lot of work to increase the stability of these apparatus to ensure repeatable, highly sensitive data was acquired for analysis.
In scanning transmission electron microscopy (STEM), the microscope lenses can be adjusted to create a focused convergent electron beam to probe the sample that is being studied.
The sample is then scanned by this concentrated probe, and numerous signals are gathered point by point to create a picture.
The samples in STEM are commonly stained with uranyl acetate. Uranyl acetate causes a high electron density in the sample, which enhances image contrast.
Electron energy loss spectroscopy (EELS) can be performed in a STEM. A beam of electrons is accelerated to energies in a STEM that is generally between 100 keV and 1 MeV. A sample consisting of a thin piece of material, typically less than 50 nm thickness, is passed through by an electron beam. The operator can discover information about the sample through the interaction of the beam with the sample, including the chemical elements present, stoichiometry, energy levels, electronic structure, and more.
The inelastic interaction between the electrons from the primary beam and the electrons from the sample is the main focus of the EELS method.
The primary electrons utilized in EELS typically experience energy losses between 0 eV and 3 keV. The dispersive characteristics of a homogeneous magnetic field on the charged electrons in an EEL spectrometer are used to calculate this energy loss.
Experimental Results
The results produced by applying STEM and EELS to the different silicon (Si) point defects in monolayer graphene were analyzed by the research team.
In the energy-loss area of about 100 meV, the Si-C4 defect generated stronger vibrational signals when compared to the Si-C3 defect. The two defect arrangements of a single impurity show different vibrational modes.
The researchers conducted an atom-by-atom examination concentrating on the carbon atoms around the impurities to assess the extended effect of the two defects and discovered that the defects only have a noticeable impact on the closest neighboring carbon atoms. The carbon atoms that are near to one other in graphene virtually act normally.
Surprisingly, in these two types of Si point defects with various bonding arrangements, the researchers discovered the low-energy phonon peak's variations in frequency for the
closest neighboring carbon atoms. Combining this finding with density-functional theory calculations, the research team demonstrated that the two defects' vibrational modes, which are principally controlled by local configurational symmetry, are responsible for the distinct vibrational signals of Si and the closest neighboring C atoms.
The research team also looked at a different defect with a significantly smaller mass—the studied nitrogen, in the defect form N-C3. Contrary to Si-C3, the high-frequency peak, which is responsible for the majority of the optical phonon modes, is where the vibrational variation is most prominently seen. The extension of the closest neighbor was observed to persist.
Outlook
In order to gain insight into the defect-induced physics in two-dimensional materials, this work has achieved accurate determinations of the vibrational characteristics of point defects in graphene and boosted the single-atom vibrational spectroscopy's capacity to measure the strength of chemical bonds. Advancements in nanophotonics can benefit from the results obtained in this study to engineer precise material characteristics.
More from AZoOptics: How is Mass Spectrometry Used in Microplastic Pollution Research?
References and Further Reading
Xu, M., Bao, DL., Li, A. et al. Single-atom vibrational spectroscopy with chemical-bonding sensitivity. Nat. Mater. (2023). https://doi.org/10.1038/s41563-023-01500-9
Hage, F. S., Radtke, G., Kepaptsoglou, D. M. et al. (2 more authors) (2020) Single-atom vibrational spectroscopy in the scanning transmission electron microscope. Science. pp. 1124-1127. ISSN 0036-8075
Chinese Academy of Sciences. (March 23, 2023). Single-atom vibrational spectroscopy now sensitive at level of chemical bonds. [Online] ChemEurope.com. Available at: https://www.chemeurope.com/en/news/1179925/single-atom-vibrational-spectroscopy-now-sensitive-at-level-of-chemical-bonds.htm
Disclaimer: The views expressed here are those of the author expressed in their private capacity and do not necessarily represent the views of AZoM.com Limited T/A AZoNetwork the owner and operator of this website. This disclaimer forms part of the Terms and conditions of use of this website.