With high speed and accuracy, the laser flash method has become a popular and effective tool in thermal analysis for evaluating materials' thermal properties, such as thermal conductivity and diffusivity. As a result, researchers can better understand the heat transfer characteristics of materials and the thermal responses of various materials.
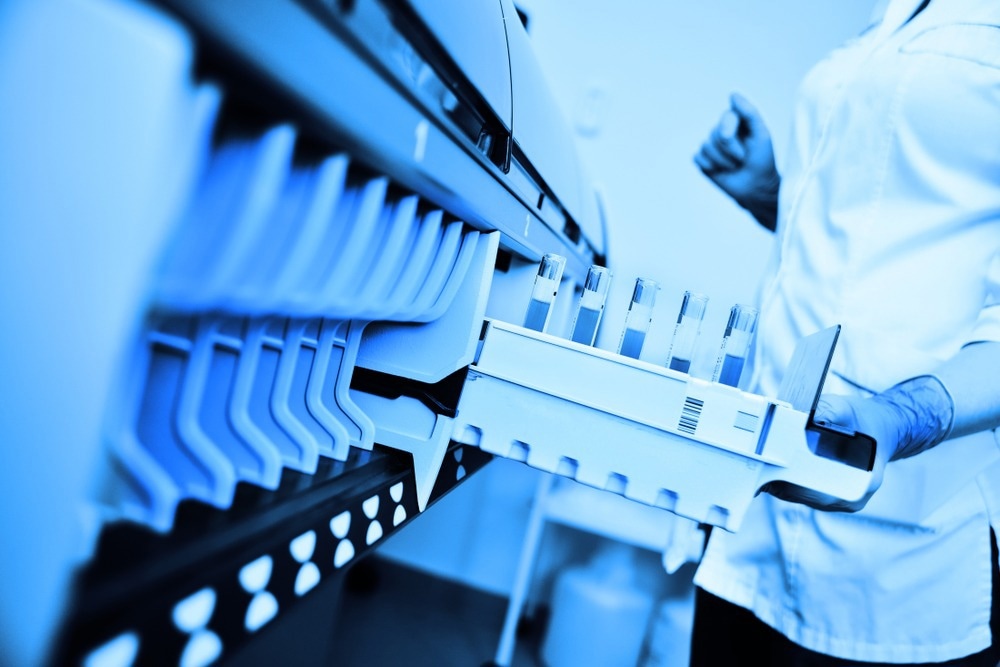
Image Credit: Evgeniy Kalinovskiy/Shutterstock.com
Background and History
Thermal analysis is becoming increasingly significant in modern industries. For example, it plays a crucial role in developing heat-dissipating materials for modern miniaturized electronics, thermoelectric and insulating materials for energy efficiency, protective coatings for turbine blades, and ensuring the safe functioning of nuclear power plants.
The laser flash method, introduced by Parker et al. in 1961, has grown to be one of the most popular techniques in thermal analysis. The method determines the thermal properties of a sample by analyzing its thermal response after exposing it to a short burst of radiant energy.
How is Laser Flash Method Used for Thermal Analysis?
The laser flash method in thermal analysis involves exposing the front side of the sample to a brief burst of laser energy, causing the internal temperature to rise and creating a thermal gradient.
The heat flow at the rear side of the sample is then measured until the sample reaches thermal stability. At thermal stability, a time-temperature graph is plotted to determine thermal diffusivity.
Parker et al. derived a formula to calculate the thermal diffusivity (α) using the half-rise time (t*), which is the time taken by the temperature to reach half of its maximum value:
α=0.1388 Δx2/t*
Δx = the material thickness. The thermal conductivity (k) is calculated from the following equation:
k=α.ρ.cp
cp is the specific heat, and ρ is the mass density.
Advantages of the Laser Flash Method
The laser flash method differs from the heat-flow-meter and guarded-hot-plate methods as it is based on an unsteady temperature distribution rather than a steady state.
The laser flash method is preferred for evaluating ceramics and metals at high temperatures. It can determine thermal conductivities from 0.1 W/(mK) to over 1000 W/(mK), across a broad temperature range of -150 °C to over 2500 °C.
It offers fast, nondestructive evaluation and needs a small sample size (6 to 18 mm in diameter) ranging from low to high conductivity. Although it requires precise machining, it has a relatively short testing time (a few milliseconds), which makes it ideal for testing highly conductive materials.
Drawbacks Associated with Laser Flash Method
A laser beam with an uneven energy distribution or a sample that absorbs thermal energy unevenly might provide inaccurate measurements of sample diffusivity. This impact is significantly magnified in thin films.
Heat transmission between a sample and its surroundings is unavoidable and usually happens via radiation and convection. However, it leads to fluctuations across measured temperatures, which adds complexity to mathematical modeling.
The finite pulse-time effect occurs when the laser pulse is emitted for a specific duration rather than instantly. This effect takes a long time to observe temperature changes in the sample.
LFA 1000 Laser Flash Instrument
The LFA 1000 is a popular laser flash equipment for calculating thermal conductivity, thermal diffusivity, and specific heat.
Its fast turntable enables the examination of up to six samples in a single experiment. Its three interchangeable ovens enable measurements to be taken in a temperature range of -125°C to 1600°C.
Its design separates hardware and electronics and provides an isolated area for examining radioactive materials.
Research and Development
Measurement noise caused by electromagnetic interferences or detectors adds uncertainty to the thermal assessment values. Artem Lunev and Robert Heymer published a study in Review of Scientific Instruments, in which they proposed to reduce the measurement uncertainty of laser flash analysis through a combination of nonlinear optimization techniques and finite-difference solutions. This approach allowed for a more precise determination of thermal properties despite measurement noise.
In a study published in the International Journal of Thermophysics, Simon Breuer & Frank R. Schilling proposed to improve the accuracy of thermal diffusivity measurements by taking into account the delayed response of the detector. According to the researchers, even if a finite pulse-time effect correction algorithm is used, the measurements might still contain significant systematic errors due to an additional time delay caused by the electronic components and detector.
Failing to consider this additional delay can result in errors that are just as large as the finite pulse-time effect. To reduce these errors, especially when working with thin films and materials with high thermal diffusivity, a data evaluation routine that accounts for the actual laser pulse shape and includes the effects of detector retardation is a viable solution.
Concluding Remarks
The laser flash technique effectively evaluates the thermal diffusivity and thermal conductivity of isotropic solid materials. However, when applied to materials that don't conform to ideal conditions, the results can be significantly biased, and it is important to be cautious when interpreting the data.
Therefore, it is recommended to conduct thorough testing and carefully consider the method's limitations to maximize the benefits of using laser flash for thermal analysis.
More from AZoOptics: The Use of Silicon in Optical Fibers and Optoelectronics
References and Further Readings
Breuer, S., & Schilling, F. R. (2019). Improving thermal diffusivity measurements by including detector inherent delayed response in laser flash method. International Journal of Thermophysics, 40, 1-17. https://doi.org/10.1007/s10765-019-2562-9
Linseis. (2022). LFA 1000 High performance Laser Flash measurements. [Online]. Linseis. Available at: https://www.linseis.com/en/products/thermal-conductivity-instruments/lfa-1000/ (Accessed on 29 January 2023)
Lunev, A., & Heymer, R. (2020). Decreasing the uncertainty of classical laser flash analysis using numerical algorithms robust to noise and systematic errors. Review of Scientific Instruments, 91(6), 064902. https://doi.org/10.1063/1.5132786
Mbaga, C. (2021). The Benefits and Limitations of Laser Flash Analysis for Determining Thermal Conductivity. [Online]. C-Therm. Available at: https://ctherm.com/resources/newsroom/blog/the-benefits-and-limitations-of-laser-flash-analysis-for-determining-thermal-conductivity/ (Accessed on 29 January 2023)
Parker, W. J., Jenkins, R. J., Butler, C. P., & Abbott, G. L. (1961). Flash method of determining thermal diffusivity, heat capacity, and thermal conductivity. Journal of applied physics, 32(9), 1679-1684. https://doi.org/10.1063/1.1728417
Souza, R. R., Faustino, V., Gonçalves, I. M., Moita, A. S., Bañobre-López, M., & Lima, R. (2022). A review of the advances and challenges in measuring the thermal conductivity of nanofluids. Nanomaterials, 12(15), 2526. https://doi.org/10.3390/nano12152526
Taylor, R. E., Gembarovic, J., & Maglic, K. D. (2002). Thermal diffusivity by the laser flash technique. Characterization of Materials. https://doi.org/10.1002/0471266965.com102
Tec-Science. (2020). Laser-Flash method for determining thermal conductivity (LFA). [Online]. Tec-Science. Available at: https://www.tec-science.com/thermodynamics/heat/laser-flash-method-for-determining-thermal-conductivity-lfa/ (Accessed on 29 January 2023)
Disclaimer: The views expressed here are those of the author expressed in their private capacity and do not necessarily represent the views of AZoM.com Limited T/A AZoNetwork the owner and operator of this website. This disclaimer forms part of the Terms and conditions of use of this website.