Images of two-dimensional ice captured using atomic force microscopy (AFM) demonstrate a previously unseen growth mechanism.
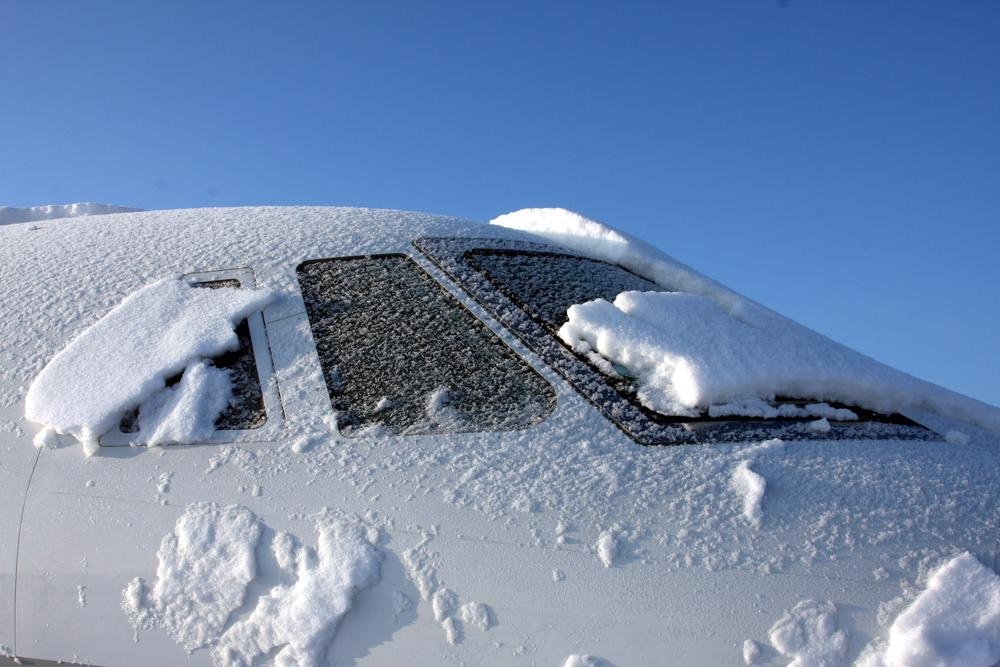
Image Credit: VT750/Shutterstock.com
Thin layers of ice forming on windowpanes and windshields on cold days are common. The underlying physical mechanism that leads to the formation of 2D ice has not been well understood. Recent advancements in AFM techniques have allowed scientists to study this phenomenon in more detail. AFM results obtained from experiments and theoretical simulations could assist in developing better device technology in materials science, chemistry, biology and atmospheric science.
Structure of Thin Ice
Significant attention has been paid to studying the behavior of water and ice in the past. Ice formation at the interface of solid surfaces has been specifically investigated with great interest. Ice growth in two-dimensional (2D) structures provides an ideal platform for analysis. 2D ice is layers of ice that are only several water molecules thick.
The structure of naturally occurring ice has a hexagonal shape forming a six-sided structure. One plane of hexagonal ice is similar to 2D ice. In the planer layout, ice can have two types of edges. Most natural ice has "zigzag" edges. The second type is called the "armchair" edge.
AFM is a powerful instrument for studying the structure and dynamics of ice. Typically, 2D bilayer hexagonal ice is grown on a metallic surface in an experimental environment. For example, on a gold (Au) substrate, before being probed by an AFM.
Atomic Force Microscope
AFM uses a nanoscale tip that hovers over or drags across a sample surface to produce a topological image. The tip is attached to a small cantilever which forms a spring.
When the tip experiences a force from the sample, the attached cantilever bends proportionally to the force.
A laser beam directed at the back of the cantilever is deflected by the contracting and stretching cantilever. A photodiode detects the laser signal, which produces an image, as shown in figure 1 (a).
Whether the nanoscale tip touches the sample surface or not depends on the operational mode of the AFM.
There are various contact and non-contact operational strategies available on an AFM. The choice of operating mode depends on the objective of the experiment.
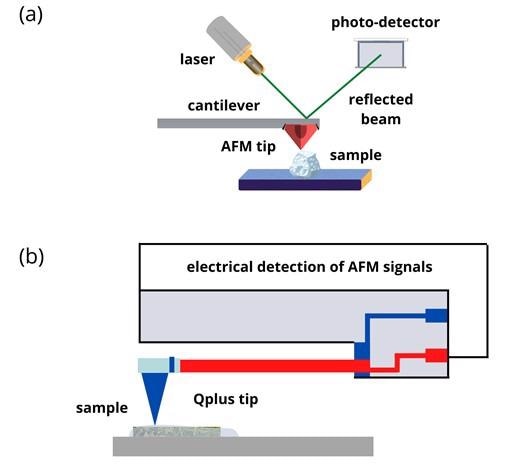
Figure 1: (a) Schematic illustrations showing basic principles of AFM. (b) AFM with Qplus probe. Image Credit: Ilamaran Sivarajah
Qplus AFM
The Qplus sensor is an innovative variation in AFMs that uses a self-sensing cantilever based on quartz.
Quartz exhibits a piezoelectric property that can generate an electrical charge in response to applied mechanical stress.
A quartz tip negates the need for laser-based detection in AFMs.
QPlus AFMs use electrical detection (see figure 1 (b)) and are better suited for studying phase change dynamics, such as ice formation, where traditional laser-based detection is challenging.
The mechanical properties of quartz make them very sensitive to minor temperature variations, and their stiffness makes them ideal nanoscale tips that can withstand higher degrees of wear.
Functionalized Tips
QPlus-based tips functionalize different materials to suit various experimental requirements.
Tip functionalization enhances molecular imaging with AFMs. A series of chemical steps are followed to attach molecules to the tip of the AFM. For example, carbon monoxide (CO) is widely used in functionalized tips.
Qplus AFM on Ice
2D ice imaged with Qplus AFM using functionalized tips has provided unprecedented resolution.
Since the high-order electrostatic forces between the ice and the AFM tip are fragile, and because the Qplus tip has high flexibility, the tip interacts weakly with the water sample.
The weak interaction reduces the damage that occurs to the fragile edge structures of the ice.
Individual hydrogen and oxygen atoms of the water molecule within the unstable intermediate edge states have been resolved by Qplus AFM.
Furthermore, armchair edges of 2D ice have been observed to stabilize and follow reaction pathways that have not been observed before.
Outlook of Studying Thin Ice with AFM
The new information acquired using Qplus AFM on thin ice helps develop lubricative materials and devices that can remove ice from surfaces.
A practical example where ice removal is critical is in structures such as wind turbines, which cannot function if covered in ice.
The Qplus AFM method is expected to expand our knowledge of the growth mechanisms of other 2D materials and how 2D ice becomes 3D.
References and Further Reading
Ma, R., Cao, D., Zhu, C. et al. (2020) Atomic imaging of the edge structure and growth of a two-dimensional hexagonal ice. Nature 577, 60–63 https://doi.org/10.1038/s41586-019-1853-4
Bart Weber, Yuki Nagata, Stefania Ketzetzi, Fujie Tang, Wilbert J. Smit, Huib J. Bakker, Ellen H. G. Backus, Mischa Bonn, and Daniel Bonn. (2018) Molecular Insight into the Slipperiness of Ice. The Journal of Physical Chemistry Letters.9 (11), 2838-2842 https://doi.org/10.1021/acs.jpclett.8b01188
Franz J. Giessibl. (2019) The qPlus sensor, a powerful core for the atomic force microscope. Review of Scientific Instruments. 90, 011101. https://doi.org/10.1063/1.5052264
Bruker Nano Surfaces (2011) Tip Functionalization Approaches for Molecular Recognition Measurements. [Online] AZoNano.com. Available at: https://www.azonano.com/article.aspx?ArticleID=2801
Disclaimer: The views expressed here are those of the author expressed in their private capacity and do not necessarily represent the views of AZoM.com Limited T/A AZoNetwork the owner and operator of this website. This disclaimer forms part of the Terms and conditions of use of this website.