The odorless and colorless nature of many gases means that gas leaks can quickly go unnoticed. In many industries that handle or produce gaseous species, it is crucial to use detectors capable of quantifying the concentrations of known gaseous species in a target atmosphere. For example, common types of gas analysis might involve monitoring oxygen levels to avoid asphyxiation risks or detecting the presence of toxic gases such as carbon monoxide.
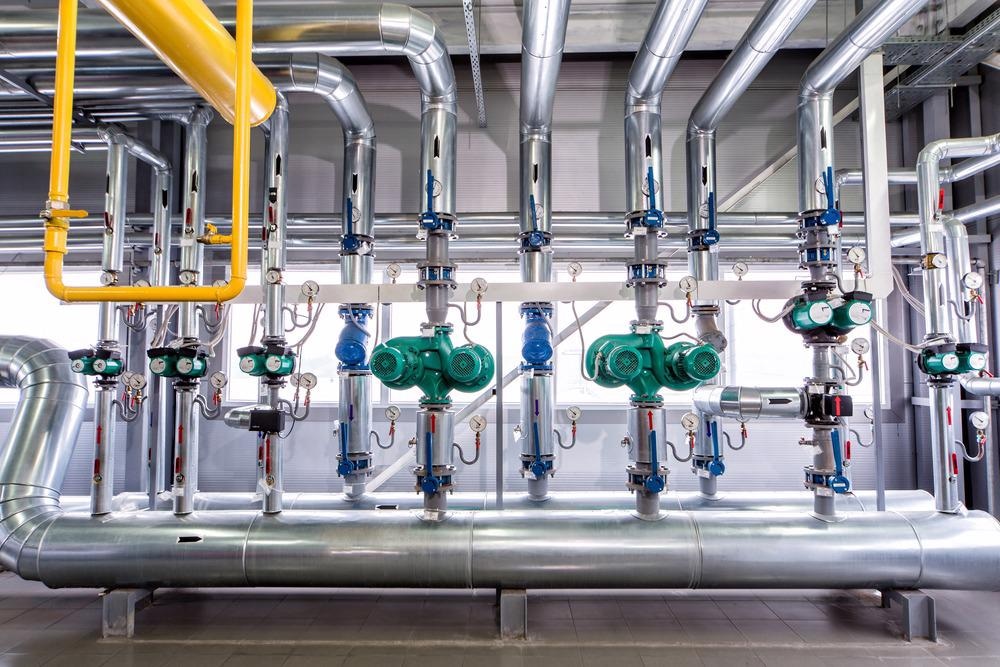
Image Credit: Alexxxey/Shutterstock.com
Gas analysis procedures are also used in many industries such as the automotive industry for assessing the composition of exhaust gases. This can be to check for regulatory compliance or to evaluate the performance and properties of different potential fuel types.1
Gases can be very challenging to analyze as they have very low number densities. For many gas analysis applications, the detection sensitivity of the analyzer needs to be capable of detecting concentrations in the parts per trillion. This is known as trace gas analysis and plays a vital role in detecting dangerous gases and also for atmospheric composition studies, including trying to understand how certain environmental conditions influence the rates of formation of particular chemical species.2
Laser Spectroscopy
There are several methods for gas analysis, including mass spectrometry techniques. Laser spectroscopy is one of the most widely utilized techniques, particularly for trace gas detection.3
Laser-based spectroscopy methods use a laser as a light source to perform spectroscopy measurements to quantify and potentially identify different gas species.
Absorption-based methods that rely on known wavelength-dependent photoabsorption cross-sections to use the Beer-Lambert law to calculate concentrations are prevalent. In such experiments, the amount of absorption of the incident laser light by the gaseous sample over a known path length is used to calculate the concentration.
However, one of the challenges for gas analysis using absorption is that the signal levels are low, making it challenging to obtain sufficient signal-to-background levels that can accurately quantify gas levels.
Gases generally have very low number densities - the number of atomics or molecules per unit volume – so even a strongly absorbed wavelength will often only cause a slight reduction in the incident light intensity.
Several experimental approaches have attempted to increase signal levels for gaseous samples. One has been the development of cavity ring-down-based methods, where the path length of the gaseous sample is increased dramatically by creating an optical cavity to hold the sample.4
At the two ends of the optical cavity are two mirrors, which are nearly totally reflective. On each bounce of the light through the cavity, more is absorbed by the sample, and only a very small proportion will pass through one of the end mirrors to be detected. After many bounces through the cavity, effective path lengths of even kilometers can be achieved in relatively compact experimental set-ups.
Impact on Gas Analysis
The development of laser sources in a range of wavelength regions capable of producing high light intensities has significantly impacted gas analysis.5 Many gaseous species have sharp resonances and spectroscopic transitions with narrow linewidths resulting in considerably more sample absorption.
The availability of tunable wavelength lasers meant that the resonances of molecules could be exploited to improve the signal-to-noise in gas analysis experiments by enhancing the absorption by the sample.
The energetic positions of these resonances, particularly for vibrational transitions in the infrared, can give a fingerprint for identifying the molecular species.
Many gas analyzers and sensors exploit molecular resonances to enable selectivity in gas detection in optical absorption methods and techniques such as photoacoustic spectroscopy, another powerful laser-based spectroscopy approach to gas analysis.6
Improvements in light source and detector technologies have meant gas analysis can be performed on trace gas levels with sufficiently short acquisition times for real-time analysis and monitoring.
Tunable diode lasers using lock-in amplifiers have been critical in advancing the applicability of gas analysis to a broader range of molecular species with spectroscopic transitions that necessitate the use of different wavelengths.
Many challenges lie in designing gas analyzers that can cope with complex environments, including exposure to corrosive or highly variable conditions, such as gas analyzers in mines or refineries.
The development of more portable devices for measuring gases in more complex environments is needed. This includes dissolved gas analysis to analyze the gas content of liquid samples for environmental work and blood analysis and medical applications.
References and Further Reading
- Fattah, I. M. R., Masjuki, H. H., Liaquat, A. M., Ramli, R., Kalam, M. A., & Riazuddin, V. N. (2013). Impact of various biodiesel fuels obtained from edible and non-edible oils on engine exhaust gas and noise emissions. Renewable and Sustainable Energy Reviews, 18, 552–567. https://doi.org/10.1016/j.rser.2012.10.036
- Galli, I., Bartalini, S., Borri, S., Cancio, P., Mazzotti, D., Natale, P. De, & Giusfredi, G. (2011). Molecular Gas Sensing Below Parts Per Trillion : Radiocarbon-Dioxide Optical Detection. Physical Review Letters, 107, 270802. https://doi.org/10.1103/PhysRevLett.107.270802
- Materazzi, S., Gentili, A., & Curini, R. (2006). Applications of evolved gas analysis Part 2 : EGA by mass spectrometry. Talanta, 69, 781–794. https://doi.org/10.1016/j.talanta.2005.12.007
- Wheeler, M. D., Newman, S. M., Orr-Ewing, A. J., & Ashfold, M. N. R. (1998). Cavity ring-down spectroscopy. J. Chem. Soc., Faraday Trans., 94(3), 337–351. DOI: 10.1039/A707686J
- Nwaboh, J. A., Desbois, T., Romanini, D., Schiel, D., & Werhahn, O. (2011). Molecular Laser Spectroscopy as a Tool for Gas Analysis Applications. International Journal of Spectroscopy, 568913. https://doi.org/10.1155/2011/568913
- Hanyecz, V., Mohacsi, A., Pogany, A., Varga, A., Bozoki, Z., Kovacs, I., & Sazbo, G. (2010). Vibrational Spectroscopy Multi-component photoacoustic gas analyzer for industrial applications. Vibrational Spectroscopy, 52, 63–68. https://doi.org/10.1016/j.vibspec.2009.10.004
Disclaimer: The views expressed here are those of the author expressed in their private capacity and do not necessarily represent the views of AZoM.com Limited T/A AZoNetwork the owner and operator of this website. This disclaimer forms part of the Terms and conditions of use of this website.