Atomic force microscopy (AFM) is an extremely high-resolution type of scanning probe microscopy, in which a probe attached to a cantilever is scanned across a sample’s surface to detect atomic forces that reveal surface topography in three dimensions at nanometer levels of scale. A new AFM technique proposes to remove the cantilever step in favor of a flexible substrate bed beneath an array of probes.
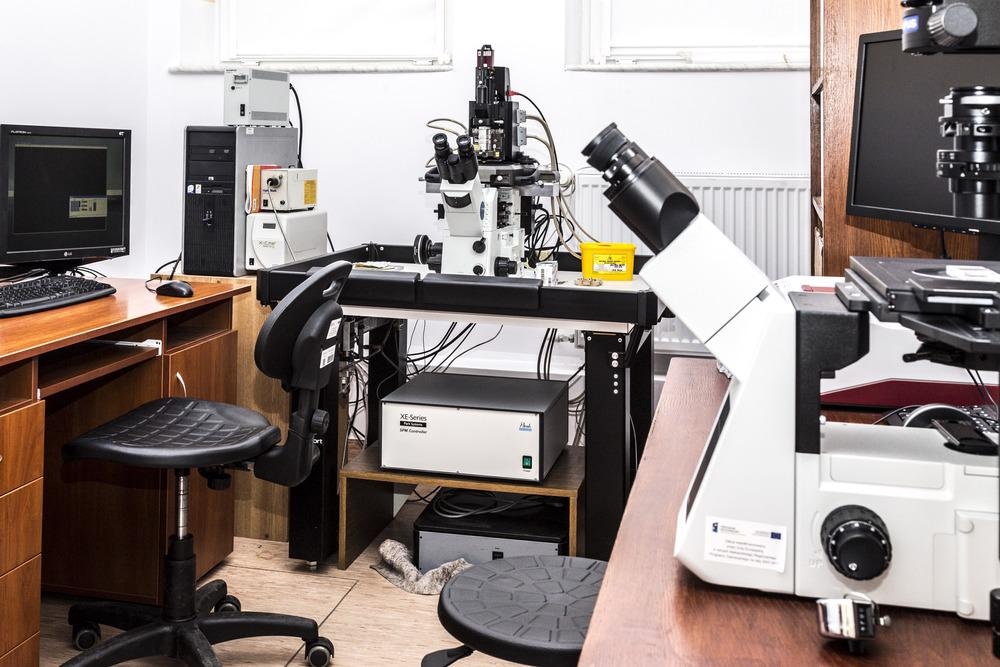
Image Credit: dominika zara/Shutterstock.com
The Role of Atomic Force Microscopy
AFM, invented in 1986, has become ever more popular in the succeeding years. It is the main method used for obtaining surface topography information at the scale of nanometers and microns. It also features strongly in functional properties research.
Conventional AFM uses a sharp probe tip attached to a cantilever to scan across the surface. Atomic forces between the tip and surface cause the probe to deviate along the z-axis (vertical axis) as it is scanned over the sample. These deviations are magnified by the leverage in the cantilever and picked up with an ultra-sensitive optical detector.
Applications for AFM
AFM has seen a wide range of applications across multiple scientific disciplines. Medicine, cell biology, molecular biology, solid-state physics, semiconductor research, molecular engineering, polymer chemistry and physics, and surface chemistry have all benefited from AFM analyses.
AFM is used to identify atoms at a sample’s surface and evaluate interactions between individual atoms in solid-state physics. It also helps us to study how physical properties change as a result of atomic manipulation changing the atomic environment.
Molecular biologists use AFM to study protein complexes and their assemblies’ structure and mechanical properties. Microtubules, for example, were imaged with AFM, which could also measure their stiffness.
AFM is used in cellular biology to differentiate cancer cells from healthy cells based on their hardness. It can evaluate the interactions between cells in competitive cultures. The probe in an AFM setup is also sometimes used to indent and deform cells to study their stiffness-regulating properties.
Conducting cantilevers can also be used in AFM to scan samples’ electric potential, a measure of the quantum mechanics operating at the sub-nanometer scale on materials’ surfaces.
Conventional AFM and Limited Field-of-View
AFM is a key example of a common tradeoff in microscopy: higher resolution comes at the cost of a smaller field of view. This limitation prevents AFM from being used to study samples that have intricate hierarchical structures across their surface.
Some attempts to tackle this have arranged multiple AFM probes in an array together. But all those individual cantilevers soon get in the way of each other and are impractical on a large scale.
Further studies resolved this issue by introducing cantilever-free arrays of probes. These place scanning tips on a deforming membrane, which is placed on top of a sensitive layer. Deviations in the probes are recorded by the sensitive layer.
New Research with Massive Cantilever-Free AFM Arrays
New research identified a need to modify these cantilever-free probe arrays to massively parallelize AFM. This would significantly increase the throughput speed of all AFM tools, enabling greater field-of-view and the ability to look at nanometer-scale features and events from a new perspective.
Researchers used more than 1,000 scanning probes in a massively parallel array. They achieved this by combining cantilever-free AFM probe systems with an optical method for detecting contact between the probe and sample that was scalable without causing individual measurements to interfere with one another.
The technique required optically reflective conical probes to be placed on a relatively compliant thin film. This created a distributed optical lever that could translate the motion of the probe into an optical signal capable of precision below 10 nm in the vertical (z) axis.
With these distributed optical levers, the team measured each probe’s deformation in a parallelized scan. The result of this parallelization (massive due to the 1,088 probes arrayed in the system) was an improved throughput compared to conventional AFM imaging.
To demonstrate the new distributed optical lever technique using cantilever-free AFM in a massive parallel array, scientists imaged an area covering 5 mm with precision on the nanoscale. A vertical resolution of 9 nm and a lateral resolution of 100 nm are consistent with the performance of conventional AFM. A sample coverage area of 5 mm is groundbreaking.
This technique enables high throughput rates for AFM which will enable us to study complex structural hierarchies. Fields such as multiscale biological tissue analysis, optical metasurface characterization, and integrated circuit metrology will all benefit from the high throughput capability of distributed optical lever AFM.
The authors of the paper, published in Nature Communications in 2021, say that the 5 mm sample coverage area they achieved is by no means the limit for the new method. While the maximum size of AFM probe arrays is limited by the optical field-of-view, large-format sensors can be used to enhance this. The scientists anticipate that arrays able to cover 100 times more sample surface area are already attainable.
References and Further Reading
Cao, W. et al. (2021) Massively parallel cantilever-free atomic force microscopy. Nature Communications. https://doi.org/10.1038/s41467-020-20612-3
Geisse, N. A. (2009) AFM and combined optical techniques. Materials Today. https://doi.org/10.1016/S1369-7021(09)70201-9.
Disclaimer: The views expressed here are those of the author expressed in their private capacity and do not necessarily represent the views of AZoM.com Limited T/A AZoNetwork the owner and operator of this website. This disclaimer forms part of the Terms and conditions of use of this website.