Miniaturization, the process of making components smaller, has been key to the realization of Moore’s Law in computing. Moore’s original observation began with noticing the trend that the number of components integrated into a semiconductor circuit would double each year.1 With this doubling came the increase in computational power that means chips less than a centimeter in size can now perform calculations that would have been unachievable by room-sized supercomputers in the past.
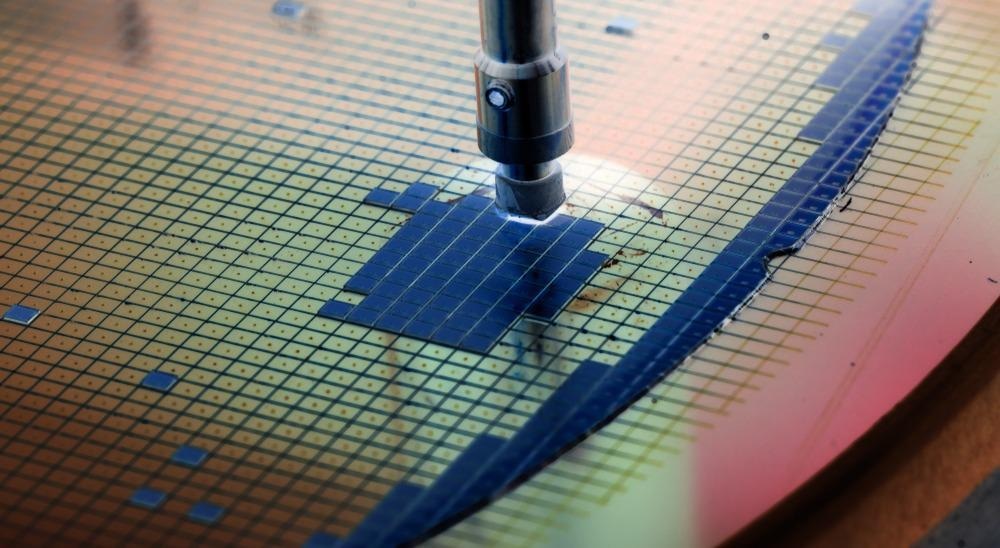
Image Credit: Macro photo/Shutterstock.com
While the continued exponential scaling of the component number of Moore’s Law appears to have reached its limit2, the huge rise and success of information technology over this period is due to the successful miniaturization of electronic components. The limits being reached now are issues with the thermal load on components and the challenge and costs associated with the machining of chips on this scale and further shrinkage will require the use of new architectures beyond just silicon components.
Increasingly, light is now being used in applications that would have previously utilized electrons and therefore been covered by the domain of electronics. Examples of this include the use of optical fibers and light transmission for faster information exchange and light is an emerging technology for use in quantum computing architectures.3 Light has the advantage of being able to travel faster than electrons and can support greater bandwidth in data transfer.
However, if photonics is truly to replace electronics, there is a need for photonic chips that can perform logic operations with photons similar to the electronic transistor. Such chips need to be sufficiently small so that they can be incorporated into devices without requiring a significant footprint.
Quantum Control
Atoms have proved an invaluable tool for quantum information. The ability to prepare atoms in a superposition of states and control this at will using laser fields has made them a popular tool in quantum computers, sensors, and devices.4 However, systems based on optical trapping of atoms require extensive amounts of cooling and often require specially designed buildings and laboratories to manage vibration levels to ensure experiments can remain interferometrically stable.
While such technologies seem to be scalable,4 these technologies are a long way from the compact photonics chip. However, recent research from the University of Illinois at Urbana-Champaign has demonstrated a way of creating simple, compact circuits that uses sound waves to control the stability of light.5
The new stabilization scheme is designed to be compatible with atomic control systems and work as an isolator to improve the stability of such experiments. Scaling down large atom-based experiments has proved challenging to date but with the use of these new isolators, smaller-scale quantum devices may now be a possibility.
Light Control
When light interacts with matter, it can undergo several processes. Those include absorption, which can promote atoms into a superposition of states needed for quantum information processes, but also other unwanted processes such as scattering. Even from well-collimated point sources such as lasers, light can be difficult to control with issues such as beam divergence and aberrations introduced by optical components, adding further challenges.
The development of optical fibers and waveguides has opened new possibilities for the control of light, including mode selection. Improvements in optics and manufacturing processes have made it possible to create resonators with minimal light loss.
A resonator is an optical cavity that allows a beam to travel in a closed path. These are used routinely in laser systems to allow for multiple passes of light over a given distance, enabling greater levels of light amplification. In waveguide-resonator systems, the resonator is coupled to a waveguide and any light that is far detuned from the resonance of the absorber will pass through the waveguide without interruption. For a critical coupling regime, there will be strong absorption of the light by the resonator.
By using a chiral waveguide-resonator system, the team created a system that completely blocks light passing in one direction, but not the other. Normally, the waveguide would be transparent to non-resonant wavelengths but they could potentially be transmitted in both directions along the guide were there to be any back reflections.
Unidirectionality of this kind has been previously achieved in waveguide-resonator systems but using magnetic fields which often requires additional bulky equipment.
The achievement of unidirectionality by switching the resonator material for a chiral substrate removes the need for such fields and is an important step towards the miniaturization of atom-based devices.
Back reflections can cause damage to optical components as well as unwanted behavior in optical components, so being able to suppress these also improves the device performance. As the system is compatible with 780 nm light, it is ideal for use with rubidium-atom-based systems that are currently being widely explored for quantum sensors.
References and Further Reading
- Mack, C. A. (2011). Fifty Years of Moore’s Law. IEEE Transactions on Semiconductor Manufacturing, 24(2), 202–207. https://doi.org/10.1109/TSM.2010.2096437
- Theis, T. N., & Wong, H. P. (2017). The End of Moore’s Law: A New Beginning for Information Technology. Computing in Science & Engineering, 19(2), 41–50. https://doi.org/10.1109/MCSE.2017.29
- Kok, P., Dowling, J. P., & Milburn, G. J. (2007). Linear optical quantum computing with photonic qubits. Reviews of Modern Physics, 79, 135–174. https://doi.org/10.1103/RevModPhys.79.135
- Cirac, J. I., & Zoller, P. (2000). A scalable quantum computer with ions in an array of microtraps. Nature, 404, 579–581.
- Sohn, D. B., Örsel, O. E., & Bahl, G. (2021). Electrically driven optical isolation through phonon-mediated photonic Autler – Townes splitting. Nature Photonics. https://doi.org/10.1038/s41566-021-00884-x
Disclaimer: The views expressed here are those of the author expressed in their private capacity and do not necessarily represent the views of AZoM.com Limited T/A AZoNetwork the owner and operator of this website. This disclaimer forms part of the Terms and conditions of use of this website.