Plant research is extremely important as it aims to address crucial issues, such as the food crisis, development of an alternative source of fuel (biofuel), novel drug development (biotechnology), environmental monitoring, and many others. Advances in microscopy, software developments, and better detection systems have revolutionized bioimaging processes. Owing to these advancements, scientists can study single living cells and three-dimensional (3D) images of cell organelles.
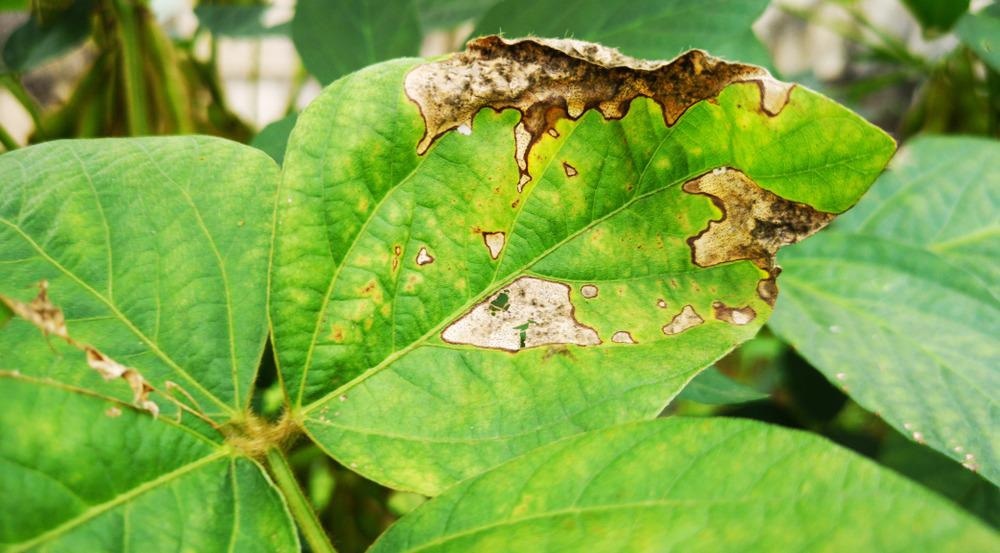
Image Credit: The natures/Shutterstock.com
Plants synthesize an enormous range of bioactive compounds and, hence, are potential sources of oils, dyes, flavors, and fragrances. Histopathological studies show where these metabolites are accumulated and other structural features of plants.
According to Dr. Raymond Wightman of the Sainsbury Laboratory (SLCU) at Cambridge University, microscopes help researchers to discover incredible details of the plants’ structure and study its function. There are different types of microscopes and each has a specific use in plant research. Some of the microscopes used are:
- Confocal microscopes
- Fluorescence microscopes
- Cryo-scanning electron microscopy (cryo-SEM)
- Atomic force microscopy
- Chemical imaging
Certain microscopes are also attached to digital imaging equipment.
Cutting-Edge Microscopes in Plants Research
To the naked eye, a typical leaf looks like a uniform green surface but a microscopic study reveals an intricate arrangement of irregular-shaped cells that are perfectly fitted together. The surface cells continue to expand and change their shape as the leaf grows.
Cell walls, which are typically 50-100 nm in width, are too small to be visualized in detail via conventional fluorescence microscopes (resolution of 200 nm). This is why scientists have combined two types of high-performance cutting-edge microscopy, namely, dSTORM and cryoSEM, to study the intact cell wall structure. This helped them identify the structure of pectin nanofilaments for the first time. These structures are a thousand times thinner than a human hair.
The cryoSEM microscopy, which is a combination of cryo (very low temperature) and scanning electron microscope (SEM), was developed at the Sainsbury Laboratory at the University of Cambridge. It took the very first images of pectin filaments. The super-high resolution light microscope confirmed the presence of pectinaceous structures in the cell wall.
Scientists revealed that these pectin nanofilaments are arranged in columns along the edge of the cell walls of plants. Before this discovery, pectin was thought to be a disorganized gel-like component present between the long cellulose fibers in the cell wall. Interestingly, researchers found that pectin nanofilamentous structures have an active role in the development of a cell shape and even cell growth, independent of pressure within the cell. This discovery is crucial as it sheds light on how plants develop complex shapes and structures that help their adaptations to continually changing environments.
These revelations could inspire the development of smart and self-expanding materials. The discovery of pectin nanofilaments in the plant cell wall has also increased plant immunity understanding.
The research team at the SLCU, led by Alexander Jones, has been developing biosensors for confocal microscopes that will enable scientists to study real-time molecules involved in plant growth. Microscopes are also used to trail living cells while they undergo cell division and other changes while developing into a fully bloomed flower. Cutting-edge microscopes are also used to study the texture of flower petals that support pollination.
Researchers used a dSTORM microscope to analyze the leaf cells of Arabidopsis thaliana (resolution of 20-40 nm) and revealed that removal of a methyl group in the pectin nanofilament could induce swelling of the filament and bring about radical expansion by approximately 40%. This swelling could lead to buckling of the cell wall, triggering the formation of unusual wavy-shaped cells.
How Do Computer Algorithms Influence Cutting-Edge Microscopic Images?
Researchers follow many strategies to create better images than those directly obtained from a microscope. For example, a method called deconvolution can fix the disorientation to 3D samples caused by the microscope. This method utilizes computer algorithms to minimize haziness and create clearer and sharper images. This method also enables accurate measurements of the sample.
Another technique is called multiview fusion, which pools numerous images and creates a 3D image and video of the studied sample.
The main limitations of these techniques are that they require extensive computing power and are time-consuming. Microscopes produce a vast amount of high-resolution data; sometimes requiring weeks or months of processing time to be usable.
A team of researchers led by Dr. Hari Shroff of NIH’s National Institute of Biomedical Imaging and Bioengineering (NIBIB) has been working to overcome these limitations of the cutting-edge microscopes.
Shroff’s team developed advanced algorithms and designed software that reduced image processing time significantly. Importantly, this method was compatible with fluorescence microscopy. This microscopy uses dyes and samples which are viewed under specific light for analysis. Scientists can view images of the specific parts of a sample and also study the interactions of different elements in the sample.
The use of a specialized computer chip for 3D rendering known as a graphics processing unit could also accelerate the processing manifold, compared to traditional processes.
Another important method that could reduce processing time is by applying a neural network, a type of artificial intelligence. In this method, scientists train the neural network to obtain clearer, cleaner, sharper, and higher resolution images.
These advancements in processing have been tested on a variety of biological samples ranging from single cells to thick tissues. Scientists demonstrated that these techniques enhanced the processing speed and quality of the images obtained from advanced microscopes that are associated with the post-processing of raw data to create 3D images.
Scientists are able to procure important biological information rapidly by considering the vast data produced by these microscopes.
References and Further Reading
Grube, K. (2021) Putting plants under microscope. [Online] Available at: https://www.cam.ac.uk/stories/plants-under-microscope
Guo, M, et al. (2020) Rapid image deconvolution and multiview fusion for optical microscopy. Nature Biotechnology. 38(11). pp.1337-1346. https://doi.org/10.1038/s41587-020-0560-x
Faster, better images from cutting-edge microscopes. (2020) National Institute of health. [Online] Available at: https://www.nih.gov/news-events/nih-research-matters/faster-better-images-cutting-edge-microscopes
University of Cambridge. (2020) Discovery of expanding pectin nanofilaments that manipulate plant cell shapes. [Online] Available at: https://www.sciencedaily.com/releases/2020/02/200227144306.htm
Haas, T.K. et al. (2020) Pectin homogalacturonan nanofilament expansion drives morphogenesis in plant epidermal cells. Science. 367 (6481). pp. 1003. DOI: 10.1126/science.aaz5103
Disclaimer: The views expressed here are those of the author expressed in their private capacity and do not necessarily represent the views of AZoM.com Limited T/A AZoNetwork the owner and operator of this website. This disclaimer forms part of the Terms and conditions of use of this website.