Hundreds of meters underwater might not seem like the natural home of a Raman spectrometer, but recent work has shown that this method's excellent analytical powers can be put to use just there.1
Raman Spectroscopy for Deep-Sea Survey and Detection" />
Image Credit: divedog/Shutterstock.com
Through the use of a titanium pressure vessel as housing, the team was able to record Raman spectra underwater up to depths of a thousand meters. Their results have contributed to the emerging trend of Raman spectroscopy in deep-sea surveying and detection.
What is Raman Spectroscopy?
Raman spectroscopy is already a workhorse technique in chemical identification and quantification for both in-situ and offline analysis. Its sensitivity to the frequencies at which the chemical bonds in a molecule oscillate make Raman spectroscopy excellent for quantitative and qualitative analysis.
The frequency of an individual chemical bond oscillation depends on both the masses of the atoms and the strengths of the bonds between them, influenced by the local chemical environment. As a result, bond frequencies provide a unique fingerprint of the molecule that can be used for identification.
The signal recorded in a Raman experiment comes from a scattering process. The sample is irradiated with a light source, typically a laser, to achieve a high light intensity. Some of the incident photons will be inelastically scattered by the sample resulting in an energy difference relative to the incident photon, known as the Raman shift. Its magnitude is proportional to the energies of specific vibrational modes in the analyte.
Raman spectroscopy is widely used in several industries for the analysis of biomolecules, materials, and chemicals.2 Its versatility and ability to retrieve quantitative and qualitative information have made it a very popular technique. Improvements in spectrometer and light source performance have meant Raman sampling times can be sufficiently short for online process analysis.
Deep-Sea Studies
The robustness of modern Raman instruments meant they have been to locations as exotic as Mars and the ocean's deepest depths.3,4 Here, it has been used to explore phenomena such as methane and dissolved sulfide concentrations, as well as in-situ analysis of natural gas hydrates that are too unstable to be brought to the surface for sampling.4
The challenge for these instruments is the need to design probes that can withstand environmental conditions like high pressure, alongside optical challenges of laser focusing and efficient signal collection over extended distances from the instrument's bulk.
Liu et al. (2021) describe some of the critical improvements to this technique, including the creation of a more compact vessel that could be deployed on a fully remotely operated vehicle. The research was published in the journal Sensors on July 27th 2021.
Through careful optimization of the setup's optical properties, the team was able to achieve a limit of detection of 0.7 nmolL-1. Although this is a low value when compared to the sensitivity of lab-based Raman setups, its value is excellent for the challenging environmental conditions the measurement was taken in.
Design Enhancements for Deep-Sea Raman
One of the key design features to achieve a decent detection limit was using a single-capsule structure instead of the multi-capsule design more commonly found in deep-sea Raman instruments.
Multi-capsule designs are used to try and balance the ease of use of the instrument against the inherently bulky size needed to withstand high-pressure operating conditions. However, such strategies require additional fiber optical coupling and collimation of the laser beam between the chambers, which introduces further losses.
A single chamber enables the use of mirrors for beam steering, which have significantly better transmission efficiencies than fiber optics. With this, the team was able to reduce the optical losses within the instrument to 9%.
One difficultly inherent to Raman spectroscopy is the choice of the optical excitation wavelength. The Raman effect decreases to the power four with increasing excitation wavelength, so shorter wavelengths are desirable to achieve higher signal levels. However, as many molecules fluoresce when excited at shorter wavelengths, moving towards UV excitation often results in sufficiently large fluorescence backgrounds; any Raman signals are obscured as a result.
The team found that, for operating in seawater, 532 nm represents the best compromise. Seawater poses its own transmission challenges at longer wavelengths, and samples such as hydrothermal and cold seep materials do not show significant fluorescence.
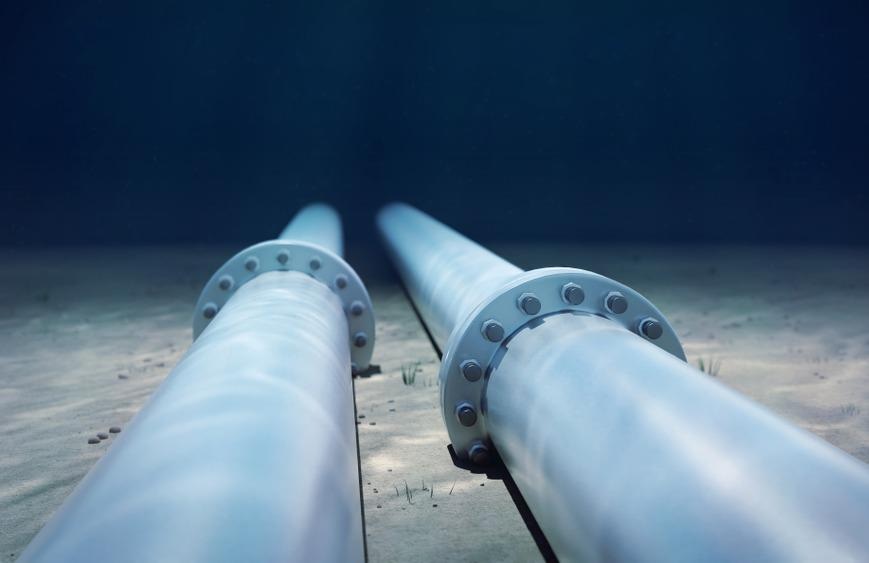
Image Credit: Vismar UK/Shutterstock.com
Future of the Industry
A lighter, more sensitive design for underwater Raman spectroscopy opens up many exciting applications for the industry. Remote operated vehicles are already used for many aspects of scientific research in the ocean and pipeline maintenance as it is not feasible to send manned craft into these conditions.5
The ability to detect a wide variety of chemical species will make deep-sea Raman an attractive option for applications such as checking for natural gas pipelines leaks or investigating regions of interest for deep-sea mining.
Some of the limitations have been overcome with the creation of smaller and more manageable instruments. Key future developments to unlocking the full potential of this technique for the oil and gas industries will be further increasing its sensitivity and reducing instrument costs.
References and Further Reading
Liu, Q., Guo, J., Ye, W., Cheng, K., Qi, F., Zheng, R., Sun, Z., & Zhang, X. (2021) Development of an Easy-to-Operate Underwater Raman System for Deep-Sea Cold Seep and Hydrothermal Vent In Situ Detection. Sensors, 21(15), 5090. Available at: https://doi.org/10.3390/s21155090.
Rostron, P., Gaber, S. and Gaber, D., (2016) Raman Spectroscopy, Review. International Journal of Engineering and Technical Research (IJETR), 6(1), pp.50 - 64. Available at: https://www.erpublication.org/published_paper/IJETR042430.pdf.
Kirkwood, W. J., Walz, P. M., Peltzer, E. T., & Brewer, P. G. (2012) A review of advances in deep-ocean Raman spectroscopy. Applied Spectroscopy, 66(3), 237–249. Available at: https://doi.org/10.1366/11-06539
Mars.nasa.gov. (2021) Scanning Habitable Environments with Raman & Luminescence for Organics & Chemicals (SHERLOC). [online] Available at: https://mars.nasa.gov/mars2020/spacecraft/instruments/sherloc/
Macreadie, P. I., et al. (2018) Eyes in the sea: Unlocking the mysteries of the ocean using industrial, remotely operated vehicles (ROVs). Science of the Total Environment, 634, 1077–1091. https://doi.org/10.1016/j.scitotenv.2018.04.049
Kirkwood, William J. (2016) Deep Sea Laser Raman – Past, Present and Future Developments for In Situ Chemical Analysis and Applications. Paper presented at the Offshore Technology Conference Asia, Kuala Lumpur, Malaysia. Available at: https://doi.org/10.4043/26505-MS
Disclaimer: The views expressed here are those of the author expressed in their private capacity and do not necessarily represent the views of AZoM.com Limited T/A AZoNetwork the owner and operator of this website. This disclaimer forms part of the Terms and conditions of use of this website.