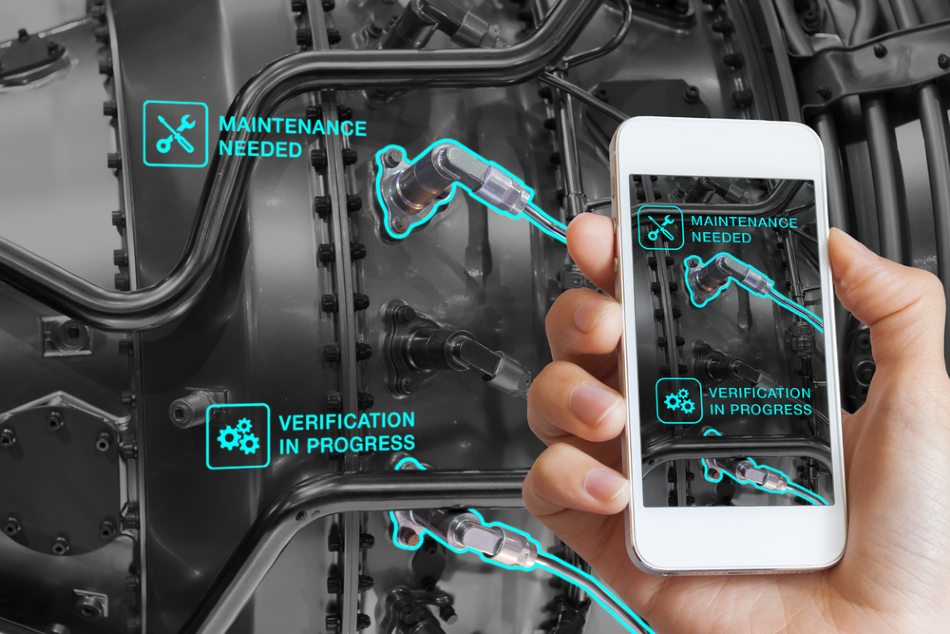
Image Credit: NicoElNino/Shutterstock.com
Augmented reality (AR) waveguides technology is being touted by many industry commentators as our best chance at realizing the promises of the future of augmented reality. Some even predict that, in the next few decades, AR will replace the keyboard, mouse or touchscreen we use to navigate the digital world today.
A new partnership between optics giant SCHOTT and the pioneers of reflective waveguides for augmented reality, Lumus, may be the beginning of the future of augmented reality.
Partnership for the Future of Augmented Reality
Reflective waveguides for augmented reality provide unparalleled functionality, flexibility and quality. However, manufacturing this technology has long been seen to be too expensive and complicated to produce viable augmented reality products at scale. The partnership between Lumus and SCHOTT is a leading step towards overcoming these difficulties.
SCHOTT is leveraging its extensive experience and world-leading manufacturing capacity to produce Lumus’s augmented reality waveguides for the mass consumer market. The economies of scale that SCHOTT can offer also means that Lumus’s pioneering product can be delivered at the lowest possible cost.
Lumus will remain responsible for the research and development of the reflective waveguides, as well as for the commercialization of the technology. SCHOTT will focus on manufacturing, making good use of their international production network with high-end optical glass melting in Germany, field-proven substrate processing lines in China, and component factory in Malaysia where the assembly line is located.
Lumus
Lumus is an Israel-based optics company with 20 years’ extensive research and industry experience in wearable displays. Their current major product is its patented reflective waveguides for augmented reality.
The technology consists of a “micro-display pod”, which projects an image into the Lightguide Optical Element (LOE), where it expands and reflects into the user’s eye. Near eye displays (NEDs) look like a pair of glasses, but the “lens” is the LOE, which is carefully engineered into reflective waveguides for augmented reality.
Lumus’s system is superior to competitor reflective waveguides for augmented reality. Its field of view (FoV) is as wide as 55°, compared to 30° in competitor products. The form factor, which is under 2 mm thick compared to 4 mm in traditional waveguides, lends designers much greater flexibility and freedom to produce NEDs that consumers will want to wear for extended periods.
Lumus’s augmented reality (AR) waveguides also boast virtual transparency, incredible brightness for daytime and outdoor usage, and bright, vivid digital images due to them projecting all colors at once.
SCHOTT
SCHOTT is a leading international technology group in the areas of specialty glass, glass-ceramics and related advanced materials. They have over 130 years of experience in optics, as well as global manufacturing and engineering know-how.
SCHOTT already produces optics for augmented refractive reality (AR) waveguides. The new partnership with Lumus adds their promising advances in reflective waveguides for augmented reality to SCHOTT’s extensive collection of cutting-edge optics.
SCHOTT’s dedicated engineering hubs in their headquarters in Mainz, Germany – as well as internationally in Switzerland and China – will also support any process innovation and metrology development that the new manufacturing process requires.
What are Augmented Reality (AR) Waveguides?
Optical waveguides are the most promising technology for enabling the future of augmented reality. This is because of their extremely low form factor and lightweight properties.
A waveguide is anything that guides waves of energy (for example, light, sound or electromagnetism) to a clearly defined endpoint. For example, fiber optic cables that carry ultraviolet light thousands of miles to transmit the data that makes up the internet do so as waveguides.
Without a waveguide, radiated energy would simply dissipate outwards from its source, its amplitude decreasing according to the inverse square law as it expands into three-dimensional space.
Optical waveguides are materials manufactured so that total internal reflection (TIR) can occur. TIR can only occur when the dielectric index of the middle layer is larger than that of the surrounding layers of material.
With TIR, images generated by an optical engine are coupled into the glass substrate, bounced back and forth inside the glass, and then coupled out of the glass into the user’s view.
One benefit of using refractive or reflective waveguides for augmented reality applications is that the micro-display or other imaging optics can be designed out of the way of the user’s view, or free movement of their face.
Reflective Waveguides for Augmented Reality
The new partnership between Lumus and SCHOTT may point to reflective waveguides for augmented reality becoming the critical enabler of the future of augmented reality.
Reflective waveguides – also known as geometric waveguides – are notoriously trickier to produce than refractive waveguides. Their manufacture involves the precise stacking of precision glass layers to create an optical combiner.
If any defect enters into any step of this complex manufacturing process, then the end result can be useless for consumers. This is where SCHOTT’s expertise and capacity will come to bear.
Read more: Using Photonics to Create Virtual Reality (VR)
The Future of Augmented Reality
Augmented reality is expected to revolutionize areas as diverse as entertainment, medicine, education, communications, manufacturing and the workplace. However, it must advance to a point where it is acceptable for mass consumer markets.
Consumers will demand low price, high resolution and high levels of comfort for wearable augmented reality technology to be worn all day. Optics need to combine external light with the projected display, and interface with simple mechanical structures (glasses frames) as well as computer processing power (probably in a nearby cell phone).
This may not be too far in the future, with Lumus and SCHOTT’s scalable mass production of reflective waveguides for augmented reality being a positive step in the right direction.
References and Further Reading
Akçayır, Murat, and Gökçe Akçayır (2017) Advantages and Challenges Associated with Augmented Reality for Education: A Systematic Review of the Literature. Educational Research Review. https://doi.org/10.1016/j.edurev.2016.11.002.
Bajarin, Tim (2017) This Technology Could Replace the Keyboard and Mouse. n.d. Time. https://time.com/4654944/this-technology-could-replace-the-keyboard-and-mouse/.
Baron, Gerald (2009) Augmented Reality – Emerging Technology for Emergency Management. Emergency Management Magazine.
Barsom, E. Z., M. Graafland, and M. P. Schijven (2016) Systematic Review on the Effectiveness of Augmented Reality Applications in Medical Training. Surgical Endoscopy. https://doi.org/10.1007/s00464-016-4800-6.
Benford, Steve, Chris Greenhalgh, Gail Reynard, Chris Brown, and Boriana Koleva (1998) Understanding and Constructing Shared Spaces with Mixed-Reality Boundaries. ACM Transactions on Computer-Human Interaction. https://doi.org/10.1145/292834.292836.
Eve, Stuart (2012) Augmenting Phenomenology: Using Augmented Reality to Aid Archaeological Phenomenology in the Landscape. Journal of Archaeological Method and Theory. https://doi.org/10.1007/s10816-012-9142-7.
Lee, Gun, and Mark Billinghurst (2012) CityViewAR Outdoor AR Visualization. Proceedings of the 13th International Conference of the NZ Chapter of the ACM’s Special Interest Group on Human-Computer Interaction. https://doi.org/10.1145/2379256.2379281.
Lock, Oliver, Tomasz Bednarz, and Christopher Pettit (2019) HoloCity – Exploring the Use of Augmented Reality Cityscapes for Collaborative Understanding of High-Volume Urban Sensor Data. The 17th International Conference on Virtual-Reality Continuum and Its Applications in Industry. https://doi.org/10.1145/3359997.3365734.
Loy Rodas, Nicolas, and Nicolas Padoy (2014) 3D Global Estimation and Augmented Reality Visualization of Intra-Operative X-Ray Dose. Medical Image Computing and Computer-Assisted Intervention. https://doi.org/10.1007/978-3-319-10404-1_52.
Mann, Steve (2015) Phenomenal Augmented Reality: Advancing Technology for the Future of Humanity. IEEE Consumer Electronics Magazine. https://doi.org/10.1109/mce.2015.2463312.
Mann, Steve, et al. (2015) Wearable Computing, 3D Aug* Reality, Photographic/Videographic Gesture Sensing, and Veillance. Proceedings of the Ninth International Conference on Tangible, Embedded, and Embodied Interaction. https://doi.org/10.1145/2677199.2683590.
Mountney, Peter, Johannes Fallert, Stephane Nicolau, Luc Soler, and Philip W. Mewes (2014) An Augmented Reality Framework for Soft Tissue Surgery. Medical Image Computing and Computer-Assisted Intervention. https://doi.org/10.1007/978-3-319-10404-1_53.
Mountney, Peter, Stamatia Giannarou, Daniel Elson, and Guang-Zhong Yang (2009) Optical Biopsy Mapping for Minimally Invasive Cancer Screening. Medical Image Computing and Computer-Assisted Intervention. https://doi.org/10.1007/978-3-642-04268-3_60.
Noelle, S. (2003) Stereo Augmentation of Simulation Results on a Projection Wall by Combining Two Basic ARVIKA Systems. Proceedings. International Symposium on Mixed and Augmented Reality.. https://doi.org/10.1109/ismar.2002.1115108.
Pair, J., J. Wilson, J. Chastine, and M. Gandy (2003) The Duran Duran Project: The Augmented Reality Toolkit in Live Performance. The First IEEE International Workshop Augmented Reality Toolkit. https://doi.org/10.1109/art.2002.1107010.
Ramo, Simon, John R. Whinnery, and Theodore van Duzer (1984) Fields and Waves in Communications Electronics. John Wiley and Sons, New York.
Shu, Jiayu, Sokol Kosta, Rui Zheng, and Pan Hui (2018) Talk2Me: A Framework for Device-to-Device Augmented Reality Social Network. 2018 IEEE International Conference on Pervasive Computing and Communications (PerCom). https://doi.org/10.1109/percom.2018.8444578.
SCHOTT (2020). Lumus and SCHOTT Enter Into Strategic Partnership. SCHOTT. https://www.schott.com/english/news/press.html?NID=com5800.
Tagaytayan, Raniel, Arpad Kelemen, and Cecilia Sik-Lanyi (2018) Augmented Reality in Neurosurgery. Archives of Medical Science. https://doi.org/10.5114/aoms.2016.58690.
Thomas, Daniel J. (2016) Augmented Reality in Surgery: The Computer-Aided Medicine Revolution. International Journal of Surgery. https://doi.org/10.1016/j.ijsu.2016.10.003.
Disclaimer: The views expressed here are those of the author expressed in their private capacity and do not necessarily represent the views of AZoM.com Limited T/A AZoNetwork the owner and operator of this website. This disclaimer forms part of the Terms and conditions of use of this website.