TIRFM makes use of an optical effect that can be adapted to observe fluorescent events occurring at the interface between two optical media of different refractive indices.
Excitation light incident upon such a boundary, travelling at an angle greater than the critical angle, undergoes total reflection. The electromagnetic field of the total internal reflected light extends into the sample beyond the interface, extending only a few hundred nanometres into the second medium of lower refractive index - essentially in the z direction.
Furthermore, this evanescent field decreases exponentially in intensity along the z-axis of penetration. Only the section of the specimen located within the evanescent field undergoes fluorescence excitation.
TIRFM is limited to the area within a few hundred nanometres of the glass/sample interface, where total internal reflection is occurring.TIRFM is an increasingly popular technique for visualizing, with high signal to background ratio, processes that occur in and around the membrane of living cells (partially due to availability of novel membrane-specific fluorophores).
In contrast to the spinning disk confocal technique which can yield rapid optical sections of approx. 1µm thickness, the excitation volume of a TIRF evanescence field extends only approx. 100nm into the sample, the intensity of which decays exponentially across this distance. This gradient can later be translated into movements (in z axis) of fluorescently abeled organelles being trafficked from and to the plasma membrane.
Since only a very thin sliver of excitation is being produced, we only detect photons that are created within that excitation volume, which has the effect of significantly improving signal to background. There are however, other sources of background photons in TIRFM that must be taken into account, and minimized where possible, as described below.
Whilst a thin excitation volume of the order of 100nm depth sounds very useful, it must be remembered that due to the nature of the TIRF effect, we cannot step this excitation plane through the cell.
TIRFM is a surface interface phenomenon, and as such is restricted to studying sample that is within 100nm or so of the glass interface of the slide. In living cells therefore, TIRFM is ideal for capturing high resolution, high Signal to Noise (S/N) kinetic series of membrane events, such as exocytosis and membrane receptor/transporter lateral dynamics. TIRFM is also a common technique for imaging single molecules dynamics, covered more thoroughly in the Single Molecule Detection area of the Biology Applications section.
Prism vs Objective-type TIRFM and Implications for Signal to Background
Two common approaches to TIRFM have evolved, often known as objective-type and prism-type, each illustrated below. The objective approach carries the key advantage that the sample is easily placed and is accessible for manipulation, e.g with patch-clamp pipette.
The approach requires that the laser be introduced through the microscope, and the angle over which TIR can be practically achieved requires use of an oil-immersion objective with a Numerical Aperture (NA) >1.4. However, the objective configuration can result in signal to background limitations, involving a combination of autofluorescence from immersion oil and stray reflections from the excitation laser which is total internally reflected back through the objective again.
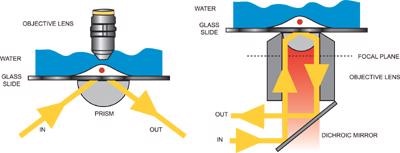
Illustration of prism-type TIRFM (left) and objective-type TIRFM (right)
The prism technique is easy to set-up, although it carries the disadvantage that the sample is located between the objective and the prism. As a consequence, more elaborate methods of sample placement and manipulation often have to be considered.
The excitation area with prism technique is potentially wider, typically up to 100µm beam waist, the illumination field is flatter and while the signal is lower with the prism technique, so is the background photon noise, considerably so since with the prism there are no stray reflections of the excitation light as with the objective TIRFM. One can also potentially avoid use of immersion oil objectives and their associated autofluorescence.
The net effect is that signal to background ratio is potentially better with the prism approach, a very important point to bear in mind when using Andor's deep-cooled iXon EMCCD camera series technology, which offers negligible instrumental noise. Given the relative advantages and disadvantages of each configuration, many TIRF experimentalists opt to set up both configurations in their laboratory, using whichever one is more appropriate to a given application.
Multiple Probes & Fluorescent Resonance Energy Transfer (FRET)
It is often of interest to dynamically image multiple different biomolecules, and their interactions within the sample volume. The TIRFM technique can readily be extended to image multiple fluorophores labels, through integration of a multi-line laser system, preferably a solid-state laser solution with Acousto-Optical Tunable Filter (AOTF) modulation. This technique can be readily adapted for FRET analysis, preferably through integration of a suitable beam splitting device on the emission side.
Combined TIRF / Widefield
A live cell microscopy set-up that enables one to switch rapidly between TIRFM and widefield epi-fluorescence microscopy, the latter for deeper penetration of the excitation into the bulk of the sample, represents a flexible and powerful approach for studying intracellular transport mechanisms.
In Dr Derek Toomre's CINEMA laboratory at Yale School of Medicine, such a combined approach is being used to visualize vesicles as they progress through the cytoplasm and eventually fuse with the cell membrane. Specially designed optoelectronic systems that take advantage of acousto-optic modulators have been assembled in several labs, acting in synchrony to oscillate illumination between TIRF and epi-fluorescent widefield.
EMCCD for TIRFM
Andor's EMCCD technology is the ideal detector for incorporation into your ultra-sensitive TIRF microscopy set-up. The extraordinary S/N offered is significantly greater than that afforded by conventional CCD cameras operating at fast readout speeds. Furthermore, since it is a fast-readout imaging device the EMCCD yields frame rates, ideally suited to dynamic acquisition of membrane events and interactions.
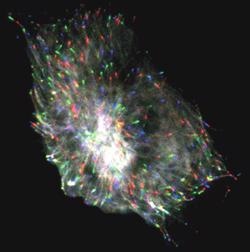
Composite triple color image of a microtubule protein (EB1-GFP) imaged with objective-type TIRFM (60x 1.45NA), incorporating the iXon DV887 back- illuminated running at 0.5 frames/sec. The different colors reveal the dynamics of the microtubules over time: frame 1 = red; frame 10 = green; frame20 = blue. Courtesy Dr Derek Toomre, CINEMA laboratory, Dept. Cell Biology, Yale University School of Medicine, CT.
Underlying all direct imaging studies of living cells or organisms, is the desire to preserve the living subject for as long as possible, through minimization of both phototoxic cell/tissue damage and also photo-bleaching of the incorporated fluorophores.
As such, the TIRF technique benefits markedly from using EMCCD detection technology, which not only exhibits the sensitivity and speed to capture, with high S/N, dynamic membrane events from within a very thin small excitation volume, but furthermore enables the laser excitation power to be attenuated. The spectral dimension is accessed either through fast switching between multiple fluorophores excitation/emission, or even splitting of the emission signal simultaneously onto different areas of the sensor.
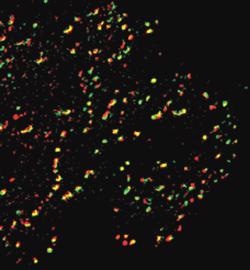
Rat kangaroo-derived Ptk2 cells were co-transfected with Dynamin2-Green Fluorescent Protein (Dyn2GFP) and Clathrin Light Chain-Red Fluorescent Protein (ClcRFP). Dyn2GFP is shown in green, ClcRFP in red. Cells were illuminated sequentially with a 488nm and a 568nm laser lines. Images were acquired by TIRFM, incorporating the iXonEM DV887 back-illuminated, driven by Andor iQ. The exposure for each channel was 150ms. Courtesy Dr Derek Toomre, CINEMA laboratory, Dept. Cell Biology, Yale University School of Medicine, CT.
Andor's iXon EMCCD camera series imaging EMCCD platform displays single photon sensitivity combined with high Quantum Efficiency (QE) at multi-MHz rapid readout speeds.
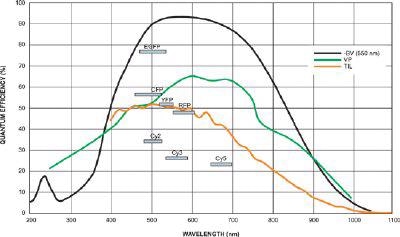
Quantum Efficiency and Fluorescent Dyes relevant to Total Internal Reflectance Fluorescence
Andor Solutions for TIRFM
The iXon EMCCD cameras are a dedicated, high-end, yet accessible ultrasensitive scientific camera platform from Andor. They are built specifically to make use of the EMCCD technology throughout all critical performance parameters and specs.
iXon Ultra and iXon3 EMCCD Camera Series
Driving the Absolute Best from EMCCD Camera Technology
Andor’s iXon design guarantees a very high level of sensitivity from a quantitative scientific digital camera, especially under rapid frame rate conditions. The latest iXon Ultra 888 camera has much better features, such as frame rates up to 3x faster and transmission of data stream through a simple plug and play USB 3.0 interface.
The well-established UltraVac™ vacuum technology from Andor has a seven-year warranty, and is important for assuring total protection as well as deep cooling of the sensor. The iXon platform range is a highly versatile and user-friendly EMCCD camera and can be optimized for various application needs with a single click using the unique OptAcquire™ feature.
Moreover, it is possible to quantitatively calibrate a signal in units of photons or electrons, either post processing or real time. This patented, pioneering technology provides automated recalibration of the linear EM gain scale coupled with anti-ageing protection. The iXon EMCCD camera is known for its reliability and product quality and has an exceptional track record with very few field failures.
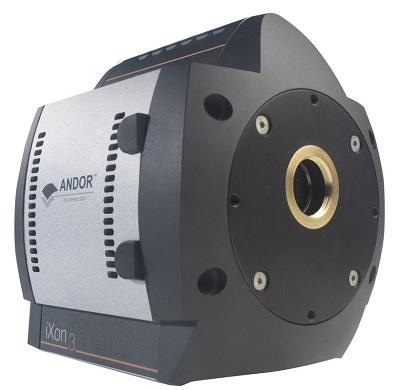

This information has been sourced, reviewed and adapted from materials provided by Andor Technology Ltd.
For more information on this source, please visit Andor Technology Ltd.