Optically active semiconductor quantum dots have high confinement potential, enabling them to interact strongly with light and hold electrons and holes. This confinement generates a multi-level system for studying quantum phenomena, making them a promising candidate for quantum computing and other emerging technologies.
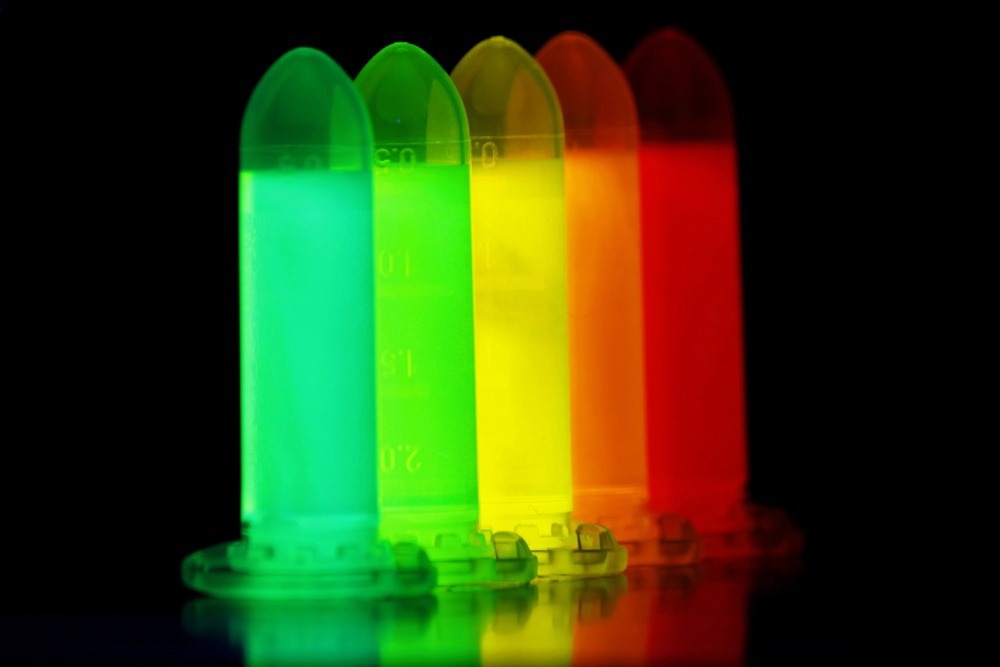
Image Credit: Dzmitry Melnikau/Shutterstock.com
Semiconductor Quantum Dots: An Overview
Quantum dots are semiconductor nanocrystals, 2-10 nanometers in diameter, that exhibit discrete electronic energy levels similar to isolated atoms. They were first proposed in the 1970s and successfully produced in the 1980s.
As the size of the semiconductor particle reduces, quantum effects come into play, which limits the energies at which holes and electrons can exist in the particle. This makes the particle's optical characteristics adjustable based on its size.
By manipulating the size of the particle, semiconductor quantum dots can be designed to absorb or emit particular wavelengths of light.
What Makes Semiconductor Quantum Dots Optically Active?
Semiconductor quantum dots are optically active only if they strongly interact with their carriers and light. This interaction is unique to quantum dots with high confinement potential that can hold both holes and electrons. Therefore, not all quantum dots are considered optically active, even if they interact with light to some degree.
Light primarily interacts with an optically active semiconductor quantum dot through transitions between the semiconductor quantum dot's conduction band and valence band or the surrounding semiconductor material.
When a short wavelength light beam hits the quantum dot, electrons absorb light energy and are excited from the valence (lower energy) to the conduction (higher energy) band. Conversely, electrons emit light when they fall from conduction to the valence band.
These interactions enable the investigation of two measurable quantities: luminescence and optical absorbance of semiconductor quantum dots. In addition, off-resonant interactions such as the Raman transitions or Faraday effect can be observed, particularly useful for manipulation and spin readout.
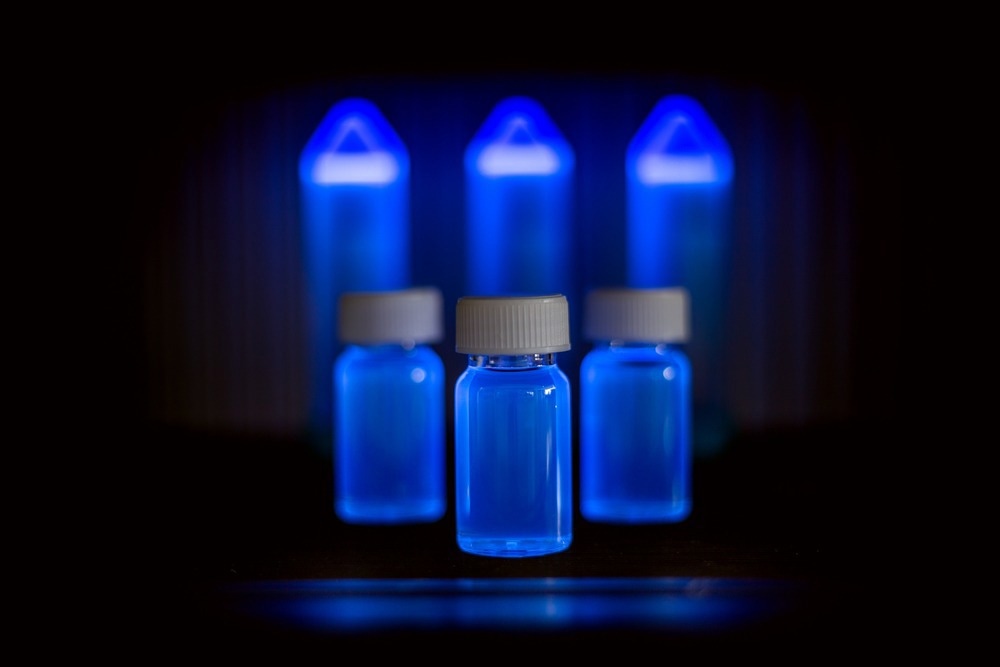
Image Credit: Tayfun Ruzgar/Shutterstock.com
Optically active semiconductor quantum dots suffer from spectral and spatial inhomogeneity; therefore, it is difficult to predict the energy and position of each quantum dot with sufficient precision. This characteristic has significant but not necessarily negative implications for quantum information processing applications.
Semiconductor Quantum Dots Fabrication Methods
Currently, self-assembled growth methods are widely used to fabricate high-quality semiconductor quantum dots. The Stranski-Krastanow growth mode effectively produces defect-free nanostructures with a narrow bandgap material in a higher-band gap matrix.
Another effective way is exploiting thickness variations that occur naturally during the epitaxial formation of thin quantum wells.
Potential Applications
Quantum Computation
Semiconductor quantum dots are a promising candidate for investigating quantum phenomena due to their multi-level system involving confined electrons and holes.
The optical active semiconductor quantum dots, such as Indium arsenide (InAs), can interfere between stationary spin and flying photonic qubits. In addition, by controlling the energy eigenstates, spin qubits trapped within a quantum dot can be manipulated.
These properties and its solid-state semiconductor nature make optically active quantum dots a promising candidate for quantum computing.
Optically Active Chiral Quantum Dots for Biological Applications
A study published in Nanophotonic Materials XII developed novel optically active chiral semiconductor quantum dot materials and analyzed their properties and biological applications.
The researcher designed chiral quantum dots with penicillamine as a stabilizer and discovered that the quantum dots synthesized with D- and L-penicillamine exhibited opposing circular dichroism spectra.
The designed chiral quantum dots identified certain biological molecules, such as amino acids and produced water-soluble, white-emitting nano-tetrapods. These nano-tetrapods displayed promising outcomes for the detection of cancer cells.
Recent Research and Development
Improving the Storage Time of Optically Active Semiconductor Quantum Dots
Efficient spin-photon interfaces are essential for quantum networks to distribute stationary quantum information as photons over long distances. Optically active semiconductor quantum dots are the most effective interface in this domain, but increasing their storage time by more than a few microseconds has proven difficult.
In a study published in Nature Nanotechnology, a team of international researchers demonstrated a simple solution to extend quantum information storage beyond a hundred microseconds.
The researchers found that using semiconductor materials with the same lattice parameter resulted in nuclei behaving in unison. This allowed for removing the extrinsic nuclear noise, resulting in a near two-order magnitude increase in storage time.
This study represents a significant advancement in preserving quantum coherence for quantum dot spin qubits, a crucial step in developing practical quantum networks and computers globally.
"Quantum dots now combine high photonic quantum efficiency with long spin coherence times. In the near future, we envisage these devices to enable the creation of entangled light states for all-photonic quantum computing and allow foundational quantum control experiments of the nuclear spin ensemble" stated Professor Mete Atatüre, co-author of the study.
Future Outlooks
The future of quantum semiconductor quantum dots looks promising. Their unique characteristics, such as high confinement potential and strong interaction with light, make them an attractive platform for a wide range of applications, and ongoing research will continue to increase their potential applications in the future.
More from AZoOptics: A Novel Non-Mechanical 3D Lidar System
References and Further Reading
Gerard, V., Govan, J., Loudon, A., Baranov, A. V., Fedorov, A. V., & Gun'ko, Y. K. (2015). Optically active quantum dots. In Nanophotonic Materials XII (Vol. 9545, pp. 43-54). SPIE. https://doi.org/10.1117/12.2187198
Gywat, O., Krenner, H. J., & Berezovsky, J. (2009). Spins in optically active quantum dots: concepts and methods. John Wiley & Sons. (Accessed on February 26 2023)
Michler, P. (Ed.). (2009). Single semiconductor quantum dots (Vol. 28). Berlin: Springer. (Accessed on February 26 2023)
Nanowerk. (2023). What are quantum dots? [Online]. Available at: https://www.nanowerk.com/what_are_quantum_dots.php (Accessed on February 26 2023)
Pooley, M. (n.d) Self-assembled quantum dots. [Online]. The University of Cambridge. Available at: https://www.sp.phy.cam.ac.uk/research/quantum-optics/qd (Accessed on February 25 2023)
The University of Cambridge. "Qubits on strong stimulants: Researchers find ways to improve the storage time of quantum information in a spin-rich material." ScienceDaily. ScienceDaily. Available at: www.sciencedaily.com/releases/2023/01/230127131213.htm (Accessed on February 25 2023)
Zaporski, L., Shofer, N., Bodey, J. H., Manna, S., Gillard, G., Appel, M. H., ... & Le Gall, C. (2023). Ideal refocusing of an optically active spin qubit under strong hyperfine interactions. Nature Nanotechnology, 1-7. https://doi.org/10.1038/s41565-022-01282-
Disclaimer: The views expressed here are those of the author expressed in their private capacity and do not necessarily represent the views of AZoM.com Limited T/A AZoNetwork the owner and operator of this website. This disclaimer forms part of the Terms and conditions of use of this website.