Recently, improvements in the speed and resolution of ultrahigh-resolution OCT has unlocked new possibilities for in-vivo OCT imaging. The possibilities for it to be a viable clinical tool for the diagnostics and monitoring treatment of corneal disease is edging ever closer to becoming reality.
Ultrafast 250 kHz imaging of the cornea at 1.5 µm resolution has now been accomplished in live subjects. This rapid process was fast enough to generate volumetric images of the cornea free of artifacts typically caused by eye movement. The speed and resolution achieved with this system enable clear determination of features of clinical and diagnostic interest including; cellular structure in the corneal epithelium; distribution of sub-basal corneal nerves and keratocytes in the stroma; mucin clusters in tear film; and even endothelial cell patterns.
With such detailed images, clinicians may one day possess much more information to help preserve the health of the cornea, the eye’s window.
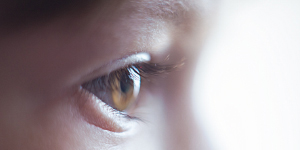
Pathological conditions of the cornea are the fourth-largest cause of blindness globally, behind glaucoma, cataract, and age-related macular degeneration.1 The difficulty that presents itself is the fact that early signs of corneal disease and any changes in the corneal tissue tend to appear at the cellular level, or in the nerve structure that is vital to its health.
Consequently, this makes signs of early deterioration undetectable using slit-lamp microscopes which are the gold standard for corneal diagnostics. In-vivo confocal microscopy (IVCM) has the necessary resolution but is restricted in its scanning speed, the field of view, and patient comfort. The capacity to detect corneal diseases earlier using a high resolution, the non-contact method would enable fast treatment and monitoring: enhancing and improving an already familiar technique could be the answer.
Over the past decade, optical coherence tomography (OCT) has been steadily advancing in its ability to deliver in-vivo corneal imaging, revealing many of the structures vital to the diagnosis of corneal pathologies. However, high-resolution volumetric images of the cornea at the cellular level have remained elusive due to imaging artifacts emanating from rapid, involuntary eye movement.
Swept-source OCT (SS-OCT) possesses the speed, though lacks the ~1 µm resolution necessary to image the corneal cellular structure. Spectral-domain OCT (SD-OCT) meets the resolution requirements but is speed restricted to <100 kHz until the recent introduction of high-speed cameras.
An SD-OCT system developed in Kostadinka Bizheva’s group at the University of Waterloo, Canada, changes everything, rapidly producing volumetric images of 0.75×0.75 mm sections of the cornea in just 2.8 seconds – sufficiently fast enough to generate clear images at the cellular level regardless of eye motion.
Furthermore, by amending the depth of focus the system is sensitive enough to map detailed volumes at every depth in the cornea. The design utilizes a Wasatch Photonics Cobra-S 800 OCT spectrometer with 200 nm bandwidth and 250 kHz line scan rate, and a femtosecond laser with stretched pulses as the source. It performs 1.5 µm axial resolution in tissue, with signal-to-noise ratio roll-off in free space of roughly 10 dB over a scanning range of 1.4 mm in depth and lateral resolution <2 µm.2
The performance of the 250 kHz OCT system was tested by imaging the central cornea of healthy, normal subjects aged 20 – 45 years, and the OCT images were compared with IVCM images acquired from the same subjects. Images were captured over the full 540-560 µm thickness of the cornea in segments, revealing clinically relevant structures in exquisite detail for every layer.
Epithelium Bound
The cornea’s outer layers are the eye’s first line of defense against the elements and infection. Here, high-speed UHR-OCT displays the cellular structure of the epithelial layer while a bright white line traces its border with the Bowman’s membrane.
Keratocytes, which assist in maintaining corneal health and promote repair, can be observed and counted in the stroma (marked with red arrows). Astonishingly, the tear film is clearly delineated and measurable on the cornea in cross-section (blue arrow), and bright spots, perhaps owing to cellular debris and mucin clusters, that can be viewed in 3D (marked by yellow arrows). Tear film data is often lost in histology and IVCM and is considerably difficult to image using other OCT methods.
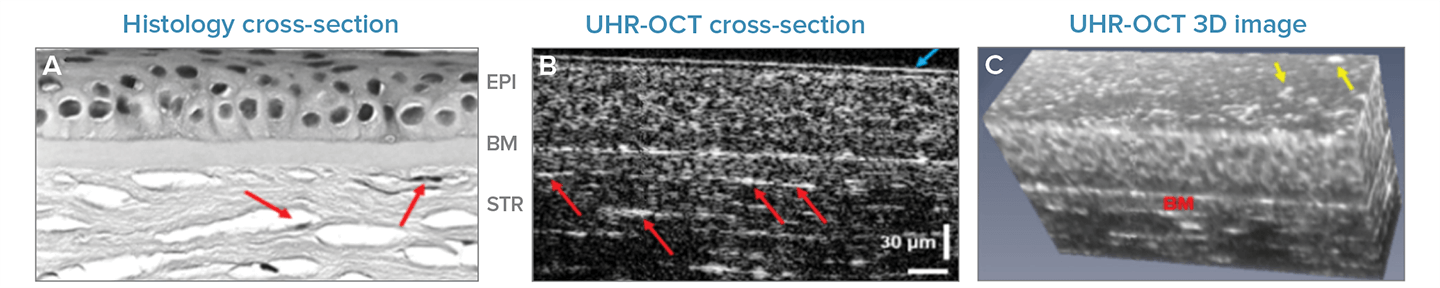
Fig 1. Anterior Corneal Layers: Corneal epithelium, Bowman's membrane, and stroma, as observed using (a) traditional histology cross-section, (b) single UHR-OCT cross-section or 'B-scan', and (c) UHR-OCT volumetric image built up from cross-sections. Adapted with permission from Tan 2018 © The Optical Society.
Every Last Nerve
Some corneal pathologies elicit changes in the length, density, and twisting of the nerves found in the basal cell layer of the epithelium, parallel to its interaction with the Bowman’s layer. The high spatial resolution and imaging rate of the UHR-OCT system enables imaging of these nerves in-vivo, illustrated here as long, reflective white lines.
The clarity of the nerves in comparison to IVCM was more than adequate to allow the research group to program a fully automated algorithm for segmentation in a subsequent study, signifying promise for monitoring and diagnostics of diseases such as diabetic peripheral neuropathy.3
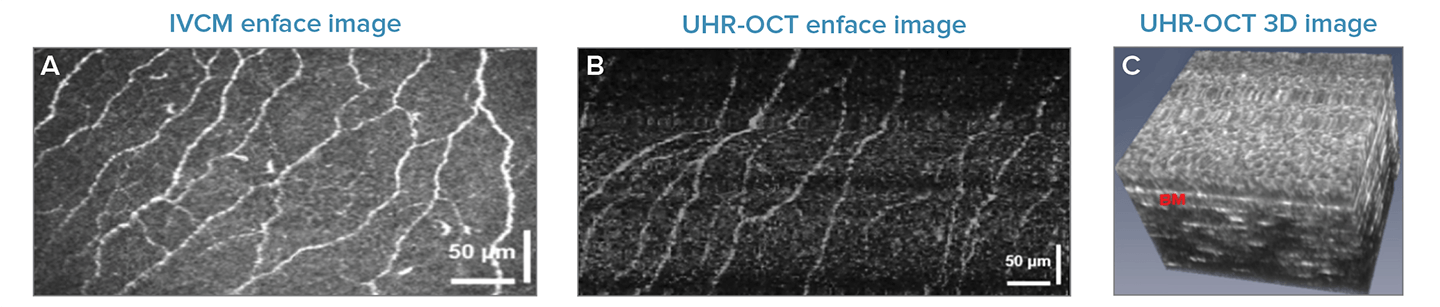
Fig 2. Corneal Nerves: Enface images or corneal nerves within the basal cell layer taken using (a) IVCM (b) UHR-OCT, and (c) a 3D UHR-OCT showing the interface of the epithelium and Bowman's membrane (bright white line) where the corneal nerves are located. Adapted with permission from Tan 2018 © The Optical Society.
Edge of the Endothelium
On the posterior edge of the cornea and close to the thick stroma rests the pre-Descemet’s layer (PDL), also known as the ‘Dua’ layer – Bizheva’s group were the first to image this layer in-vivo back in 2016.4 Under the Dua layer, the Descemet’s layer and endothelium are the last-two layers in the cornea. The precise locations of the boundaries of each layer are key to the precision and accuracy of keratoplastic laser surgery techniques.
Even at this sizable depth, the high-speed UHR-OCT system offers an appropriate resolution to clearly see each structure, including keratocytes, at the limit between the posterior stroma and PDL (bright white spots, marked by red arrows). Observing the corneal endothelium layer enface offers the possibility to see reflections from the nuclei (white spots in the UHR-OCT image), as well as their hexagonal pattern (marked in red). This is a remarkable sight given that these enface images were generated in-vivo using just 2.8 seconds of in-vivo scans.
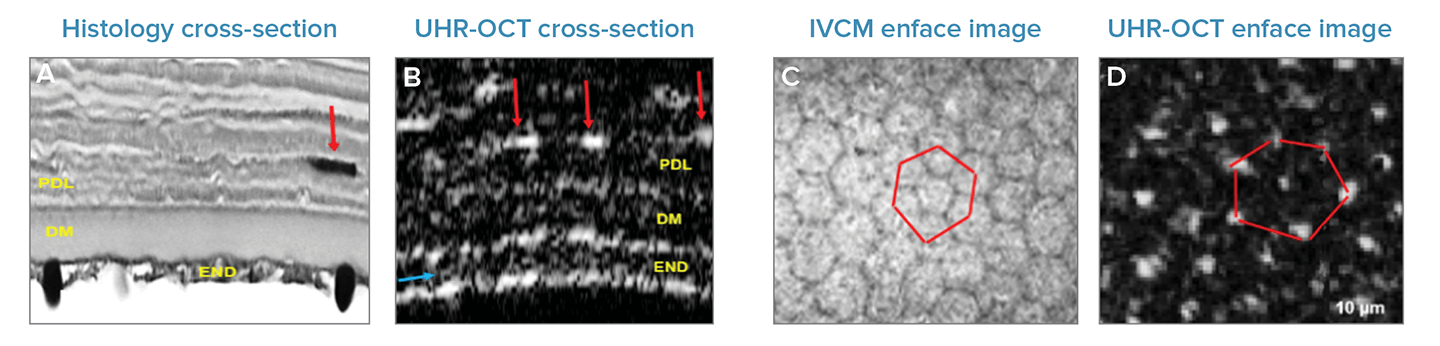
Fig 3. Cross-sectional images of the posterior stroma, PDL, Descemet’s layer, and endothelium as seen by (a) histology and (b) high-speed UHR-OCT. Cellular structure in the endothelium as revealed by (c) IVCM and (d) UHR-OCT, enface view. Adapted with permission from Tan 2018 © The Optical Society.
Conclusion
This next-generation UHR-OCT system, powered by the Cobra-S spectrometer, overcomes the typical restrictions associated with eye motion artifacts and yields an excellent, rapid acquisition rate. It also gives the fine detail necessary to see multiple corneal structures that are vital in the study, diagnosis, and treatment of corneal disease - ranging from corneal nerves, tear film, and keratocytes to the endothelial cell structure and PDL layer. In combination with automated analysis, someday this may become a powerful and influential tool in the clinic.
References and Further Reading
- WHO. “Global data on visual impairments 2010.” (2012).
- Tan, Bingyao, et al. “250 kHz, 1.5 µm resolution SD-OCT for in-vivo cellular imaging of the human cornea.” Biomedical Optics Express 9.12 (2018): 6569-6583.
- Hosseinaee, Zohreh, et al. “Fully automated corneal nerve segmentation algorithm for corneal nerves analysis from in-vivo UHR-OCT images.” Ophthalmic Technologies XXIX. Vol. 10858. International Society for Optics and Photonics, 2019.
- Bizheva, Kostadinka, et al. “In vivo imaging and morphometry of the human pre-Descemet’s layer and endothelium with ultrahigh-resolution optical coherence tomography.” Investigative ophthalmology & visual science 57.6 (2016): 2782-2787.
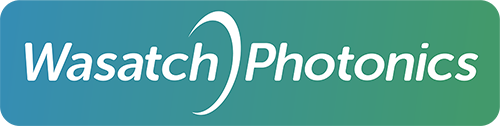
This information has been sourced, reviewed and adapted from materials provided by Wasatch Photonics, Inc.
For more information on this source, please visit Wasatch Photonics, Inc.