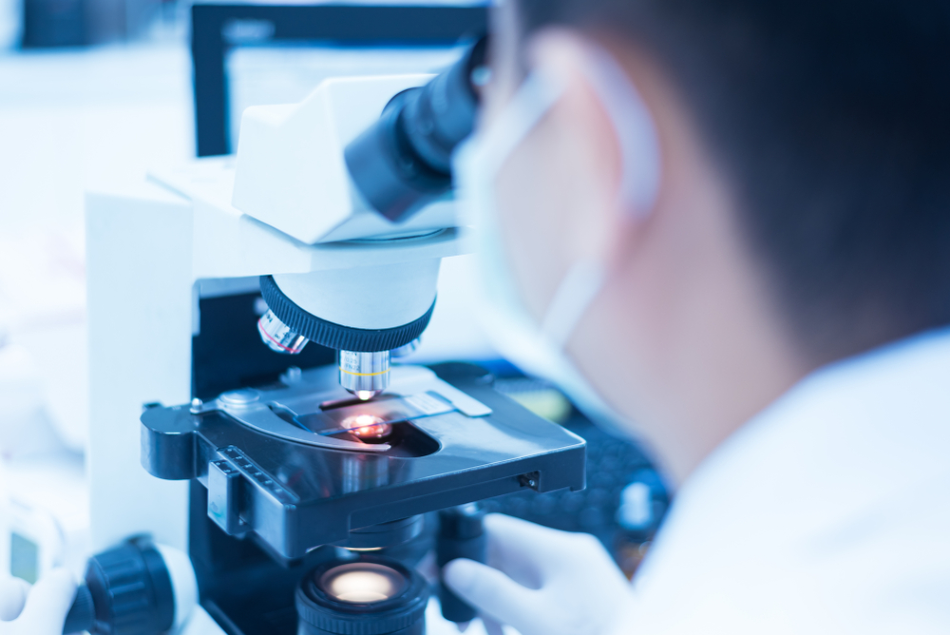
Image Credit: Komsan Loonprom/Shutterstock.com
STED Microscopy has increased the resolution of images captured by fluorescence microscopy. However, while the innovative method allowed scientists to see structures within cells in fine-grain detail, the technique suffered two major limitations, of photobleaching, and being potentially toxic to living cells.
A study published last August in the Proceedings of the National Academy of Sciences demonstrates how a team of researchers from Japan developed a method to overcome these limitations, resulting in live mitochondria being seen in unprecedented detail.
Expanding Our Knowledge of the Cell “Powerhouse”
Mitochondria, known as the “powerhouse of the cell”, convert oxygen and food sources into usable energy forms for our cells. They also have other key bodily functions, such as producing cell signaling molecules, generating body heat, regulating calcium levels (which plays an important role in signal transduction), and apoptosis (a regulated form of cell death).
While mitochondria are fundamental to the healthy functioning of human cells, it was not possible to view them in living cells, and therefore it was uncertain about how they mitigate these vital functions. Previous technology had only supported their investigation via transmission electron microscopy which can’t be used on living cells. A team of scientists in Japan recognized the potential value of developing a method to do this.
In 2014, the Nobel prize in Chemistry was won by Stefan Hell, Eric Betzig, and William Moerner for the development of Stimulated Emission Depletion (STED) microscopy. This achieved super-resolution beyond the diffraction limit that had previously hampered investigations with fluorescence microscopy. The team combated the problem with a simple but elegant solution.
Previously, fluorescence microscopy had visualized targets marked with a fluorescent biomolecule by exciting them with a laser and recording the photons emitted at the molecules return to their ground state. The method saw the photons from several fluorescing molecules being picked up by a detector using lenses, resulting in the generation a single featureless pixel from multiple sources, causing the diffraction limit.
Hell’s team overcame this by developing a two laser approach. The first laser excites the marker molecule electron, and the second laser stimulates photon emission by the excited marker molecule in the outer ring. The second laser is designed with a hole in the middle to prevent affecting the marker molecules there.
The key to the design is timing, the molecules stimulated by the outer beam are forced into their ground state before those in the center, and so delaying the measurement allows the visualization of only the molecules in the inner ring, resulting in an increased resolution that is theoretically limitless in how sharp it can make the picture.
While the team created a revolutionary technique, it suffers a major drawback. The STED beam causes molecules to degrade and stop fluorescing, known as photobleaching. These damaged markers can then become toxic to the cell, making it unsuitable for use in living cells.
The current study looked to resolve this problem to develop a method for investigating the processes occurring inside living mitochondria. This would have significant implications for the future diagnosis and treatment of human mitochondrial disease, and potentially even help develop a cure.
The Research
The team at Nagoya University, Japan, developed a way to prevent the molecules from degrading under the intense STED beam which stopped their fluorescing. They created a marker molecule that they named "MitoPB Yellow," which has a long lifetime under a STED beam. The inner membrane of mitochondria absorbs this molecule, allowing it to fluoresce for longer periods without the detrimental impact of photobleaching.
MitoPB Yellow was proven to be useful in the study of live cells when it was added to mitochondria that were then placed under conditions that cause specific structural changes to the organelles. The scientists watched the initial survival responses followed by their dying processes under the STED beam. For the first time, the team observed the structural changes of the living mitochondria which had previously only been observed in dead samples through the use of transmission electron microscopy.
The Results
The team successfully established a new fluorescent marker molecule where the intense STED beam does not cause decay, thus solving the photobleaching problem that has hindered the STED method. With this innovation, living cells can finally be viewed over relatively long periods in high resolutions below the diffraction limit. The team managed to capture 1000 sharp images of live mitochondria, which is a revolution in biological imaging.
The impact of what has been achieved by this research is likely to be profound on the field of biological imaging. Observing live mitochondria in action will enable advances in the treatment and diagnosis of human mitochondrial disease, for which there is currently no known cure.
References and Further Reading
Wang, C., Taki, M., Sato, Y., Tamura, Y., Yaginuma, H., Okada, Y. and Yamaguchi, S. (2019). A photostable fluorescent marker for the superresolution live imaging of the dynamic structure of the mitochondrial cristae. Proceedings of the National Academy of Sciences, 116(32), pp.15817-15822. https://www.pnas.org/content/116/32/15817
Weiss, P. (2014). Nobel Prizes for Super-Resolution Imaging. ACS Nano, 8(10), pp.9689-9690. https://pubs.acs.org/doi/abs/10.1021/nn505967q
Disclaimer: The views expressed here are those of the author expressed in their private capacity and do not necessarily represent the views of AZoM.com Limited T/A AZoNetwork the owner and operator of this website. This disclaimer forms part of the Terms and conditions of use of this website.