Spectroscopy is an exploratory technique employed in many scientific fields, using light in the form of a laser to examine the configuration, physical and electronic structures of matter at various scales, depending on the technique employed.
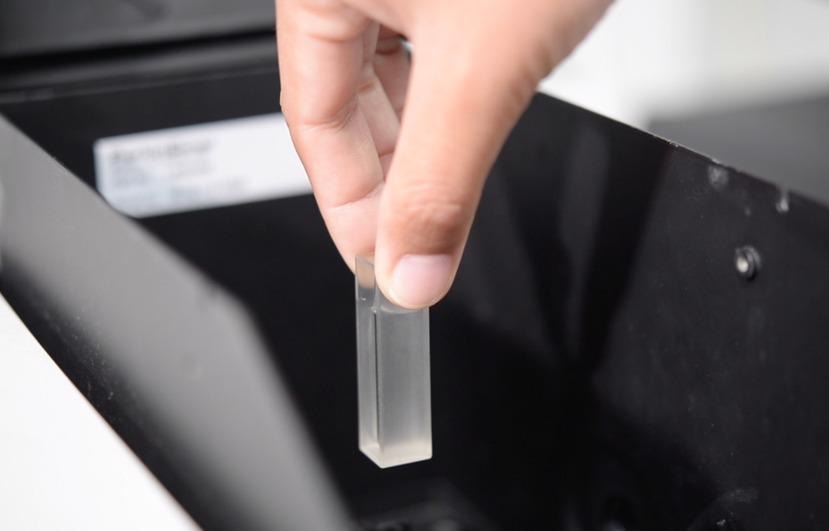
Image Credit: RabbitmindphotoShutterstock.com
Raman spectroscopy is a vital tool for advanced scientific research; it relies on the inelastic scattering of photons to measure the energy of crystal lattice vibrations in the Terahertz frequency, yielding a structural fingerprint that allows the molecule to be identified.
Having proved to be an efficient technique at determining the crystal qualities of materials as well as identifying their phase transitions, Raman spectroscopy is also used for practical applications; cocaine detection at airport security and art examination for forgery are common examples. However, related challenges limit its ability to be widely implemented.
Brillouin spectroscopy is a relatively new technique that utilizes the same basic principle, the physical scattering processes, but the information gathered is significantly different.
What is Brillouin Spectroscopy?
Brillouin spectroscopy – sometimes referred to as Brillouin-Mandelstam - is not unlike Raman spectroscopy in that it also measures the energy of crystal lattice vibrations. However, it does so in the Gigahertz range, a thousand times smaller than Terahertz.
While the smaller energies and intensity of scattered signals detected by a Brillouin spectrometer means the instrumentation is more complicated, it has already returned a plethora of new information. Scientists have been able to investigate the slightest variations in the magnetic properties of materials caused by temperature changes, stress in crystal lattices, or other provocations.
Improving the Technique
Brillouin spectroscopy is rapidly becoming an essential complementary method to Raman spectroscopy. However, estimated place it at a similar stage of development and implementation as its counterpart was 30 years ago. A recent review published in Nature Photonics on July 22nd, 2021 has offered new insights into designing and building improved spectrometers for new materials and heterostructures.
Scientists suggest the instrumentation will make it possible to detect small changes in the properties of phonons, quanta of crystal lattice vibrations, and magnons, quanta of spin waves.
Brillouin spectroscopy has in recent years become a method for studying confined acoustic phonons, phononic metamaterials, magnon propagation, and scattering. The technique has proven itself versatile and non-destructive for applications in solid-state physics as well as engineering research, says Fariborz Kargar, also of UC Riverside and co-author of the paper.
The need for determining phonon and magnon dispersions in novel two-dimensional and one-dimensional materials, nanostructures, and spintronic materials resulted in a rapid expansion of this photonic technique to new material systems.
Alexander Balandin, co-author, Professor of Electrical and Computer Engineering, University of California Riverside
Brillouin spectroscopy has in recent years become a method for studying confined acoustic phonons, phononic metamaterials, magnon propagation, and scattering. The technique has proven itself versatile and non-destructive for applications in solid-state physics as well as engineering research, says Fariborz Kargar, also of UC Riverside and co-author of the paper.
“The capabilities offered by this type of spectroscopy have already resulted in advancements in the fields of low-dimensional magnetic and non-magnetic materials and nanostructures, polymers, biological systems and imaging microscopy,” Kargar adds, “One can foresee that this technique will find even broader use in investigations where handling the small-size samples and detecting elemental excitations with small energies are essential.”
The Future for Brillouin Spectroscopy
Kargar and Balandin are leading a major project to build the world's most advanced Brillouin-Mandelstam spectroscopy system. It will have a purposely designed rotating imaging system and variable temperature stage. Special image processing algorithms will be included to locate small samples, and it will feature an exact focusing ability to be employed at different angles.
It is hoped the new instrument will allow researchers to measure the energy dispersion of phonons, magnons, and other elemental excitations in samples as small as 1 micrometer.
Efforts still need to be taken to improve Brillouin spectroscopy, including developing new instrumentation and technique refinement. Although scientists have made some exciting discoveries, it is clear that Brillouin spectroscopy has significant potential for a range of scientific disciplines.
References and Further Reading
Ober, H., (2021) Will Brillouin spectroscopy become as indispensable for science as Raman spectroscopy?. [online] Inside UCR. Available at: https://insideucr.ucr.edu/stories/2021/07/22/will-brillouin-spectroscopy-become-indispensable-science-raman-spectroscopy [Accessed 30th August 2021].
Kargar, F. and Balandin, A., (2021) Advances in Brillouin–Mandelstam light-scattering spectroscopy. Nature Photonics. Available at: https://doi.org/10.1038/s41566-021-00836-5 [Accessed 30th August 2021].
Warwick.ac.uk. (2021.) Phonons. [online] Available at: https://warwick.ac.uk/fac/sci/physics/current/postgraduate/regs/mpagswarwick/ex5/phonons/ [Accessed 30th August 2021].
Encyclopedia Britannica. (2021) Magnon | physics. [online] Available at: https://www.britannica.com/science/magnon [Accessed 30th August 2021].
Disclaimer: The views expressed here are those of the author expressed in their private capacity and do not necessarily represent the views of AZoM.com Limited T/A AZoNetwork the owner and operator of this website. This disclaimer forms part of the Terms and conditions of use of this website.