A group of researchers, guided by chemists from the University of California, Irvine, have discovered a new way in which light interacts with matter. This breakthrough has the potential to enhance the efficiency of solar power systems, light-emitting diodes, semiconductor lasers, and other technologies. The findings of the research team were recently published in the journal ACS Nano.
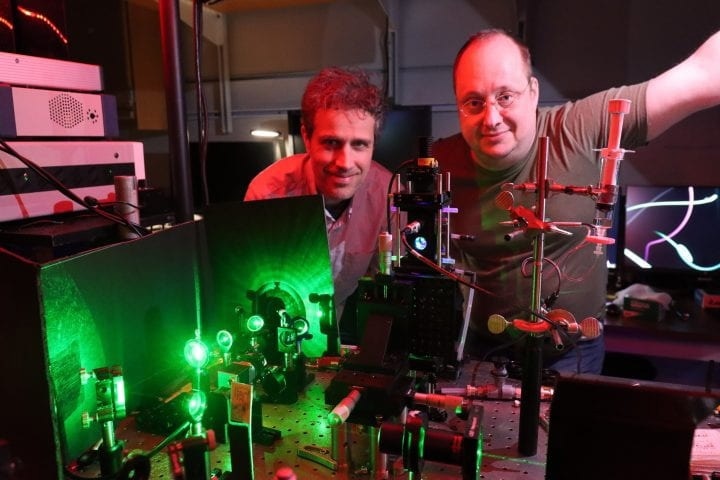
Dmitry Fishman (right) and Eric Potma, both professors of chemistry at UC Irvine, made a breakthrough discovery regarding the way light interacts with solid matter in silicon. Their work could lead to improved efficiency in solar electric systems, semiconductor lasers and other advanced optoelectronic technologies. Image Credit: Lucas Van Wyk Joel / UC Irvine
The scientists discovered that when photons are confined to nanometer-scale spaces within silicon, they gain significant momentum, similar to how electrons behave in solid materials.
Silicon is Earth’s second-most abundant element, and it forms the backbone of modern electronics. However, being an indirect semiconductor, its utilization in optoelectronics has been hindered by poor optical properties.
Dmitry Fishman, Study Lead Author and Adjunct Professor, University of California, Irvine
While silicon does not naturally emit large amounts of light, it can produce measurable light when exposed to visible light if it is in a porous or nanostructured form. This phenomenon has been known to scientists for decades, although the precise origins of the light emission have long been debated.
Fishman added, “In 1923, Arthur Compton discovered that gamma photons possessed sufficient momentum to strongly interact with free or bound electrons. This helped prove that light had both wave and particle properties, a finding that led to Compton receiving the Nobel Prize in physics in 1927. In our experiments, we showed that the momentum of visible light confined to nanoscale silicon crystals produces a similar optical interaction in semiconductors.”
To understand the origins of this interaction, it is essential to revisit the early 20th century. In 1928, Indian physicist C.V. Raman, who would go on to win the Nobel Prize in Physics in 1930, attempted to replicate the Compton experiment using visible light. He faced a significant challenge due to the substantial difference in momentum between electrons and visible photons.
Despite this obstacle, Raman's research into inelastic scattering in liquids and gases unveiled what is now known as the vibrational Raman effect. This discovery has since become foundational in spectroscopy, a critical technique in the spectroscopic study of matter, and is referred to as Raman scattering.
Our discovery of photon momentum in disordered silicon is due to a form of electronic Raman scattering. But unlike conventional vibrational Raman, electronic Raman involves different initial and final states for the electron, a phenomenon previously only observed in metals.
Eric Potma, Study Co-Author and Professor, University of California, Irvine
In their experiments, the researchers created silicon glass samples in their laboratory, varying from amorphous to crystalline states. They exposed a 300-nanometer-thick silicon film to a tightly focused continuous-wave laser beam, scanning it to inscribe an array of straight lines.
In regions where temperatures remained below 500 degrees Celsius, the process led to the formation of homogeneous cross-linked glass. Where temperatures exceeded 500 degrees Celsius, a heterogeneous semiconductor glass was produced. This "light-foamed film" enabled the researchers to examine variations in electronic, optical, and thermal properties at the nanometer scale.
“This work challenges our understanding of light and matter interaction, underscoring the critical role of photon momenta. In disordered systems, electron-photon momentum matching amplifies interaction – an aspect previously associated only with high-energy – gamma – photons in classical Compton scattering. Ultimately, our research paves the way to broaden conventional optical spectroscopies beyond their typical applications in chemical analysis, such as traditional vibrational Raman spectroscopy into the realm of structural studies – the information that should be intimately linked with photon momentum,” Fishman added.
Potma stated, “This newly realized property of light no doubt will open a new realm of applications in optoelectronics. The phenomenon will boost the efficiency of solar energy conversion devices and light-emitting materials, including materials that were previously considered not suitable for light emission.”
Jovany Merham, a junior specialist in chemistry at UC Irvine, and Sergey Kharintsev, Elina Battalova, and Aleksey Noskov from Kazan Federal University were co-authors of the study. Kazan Federal University and the Chan Zuckerberg Initiative provided financial assistance for the study.
Journal Reference:
Kharintsev, S. S., et. al. (2024) Photon-Momentum-Enabled Electronic Raman Scattering in Silicon Glass. ACS Nano. doi:10.1021/acsnano.3c12666.
Source: https://uci.edu/